G-CSF in stem cell mobilization: new insights, new questions
In this article, Bernitz and colleagues (1) utilize a lineage tracing mouse model to show that G-CSF selectively mobilizes quiescent non-dividing hematopoietic stem cells (HSC) from bone marrow (BM) to peripheral blood (PB) and that G-CSF administration does not induce HSC cycling/proliferation. Mobilized non-dividing HSC can return to BM, again without dividing. Moreover, the non-dividing HSC represent the true long-term repopulating cells, and those HSC left in BM after mobilization, while expressing phenotypic markers of HSC, do not in fact have repopulating capacity. (Summarized in Figure 1)
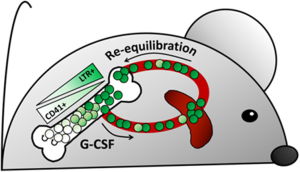
G-CSF mobilized HSC from peripheral blood (PBSC) is the most widely used source of HSC for clinical transplantation. Recently though, some questions have been raised as to whether this shift is clinically justified. PBSC mobilization, particularly with G-CSF, yields greater donor cell CD34+ numbers with faster engraftment and reduced incidence of graft failure; however, overall patient survival is not different from BM transplantation (2,3). This can be explained at least in part by increased graft versus host disease (GVHD) with PBSC transplants (2,3), but mobilized HSC may also have reduced function compared to those from the BM on a cell-to-cell basis (4).
Because G-CSF can induce proliferation within the HSC compartment (5-7) and proliferative history is inversely correlated to HSC function (8-10), Bernitz et al. hypothesized that a defect in mobilized HSC could result from G-CSF induced cell division (1). A division-tracking mouse model was employed wherein a GFP-tagged histone H2B (H2B-GFP) is incorporated into nucleosomes when expressed under the control of a tetracycline-response element, but only when the activator is available. The activator is itself controlled by a human CD34 promoter in the HSC, and can be sequestered by doxycycline treatment to halt H2B-GFP expression. After sustained doxycycline treatment, GFP intensity becomes reduced with each cell division while quiescent HSC retain high signal intensity. These label-retaining HSC (LTR-HSC) have been shown previously to contain all of the long-term repopulating HSC (LTR-HSC) potential in the BM (9).
Using this model, the authors provide several interesting findings regarding G-CSF mobilization. Significantly, the driving scientific question of this work received an unexpected and intriguing answer: G-CSF did not induce proliferation of LTR-HSC as previously thought. After a 6-week doxycycline chase, a G-CSF regimen, and a subsequent 8-week re-equilibration (with continuous doxycycline) the number of LT-HSC found in the BM was the same as that of mice that did not receive G-CSF. This indicated that the LTR-HSC did not divide in response to G-CSF. Previous reports that G-CSF induces HSC cycling were likely detecting proliferation in the CD41+ portion of the phenotypic HSC pool [defined as Lin−, Sca1+, c-kit+ (LSK), CD150+, CD48−], which was found not to contain the LTR-HSC according to label-retention in this model.
Another surprising finding from this work is that, following a G-CSF regimen, mouse BM was almost 90% depleted of LTR-HSC and the remaining BM cells were found to have very little regenerative potential in transplantation assays. This raises a clinical question; Are PBSC donors for allogeneic transplant compromised with respect to their repopulating potential following PBSC donation? In clinical PBSC collection one would expect almost all HSC in the periphery to be removed after apheresis of ≥5 blood volumes. If the current mouse model is representative, this would leave the donor with severely depleted and defective BM HSC repopulation capacity. However, it could be that the non-dividing HSC that localize to the spleen for several weeks, as seen in this model, can escape collection and are sufficient to later repopulate the donor BM stem cell pool—a question which could warrant further exploration. In this regard, studies performed in splenectomized mice might be informative.
Short-term side effects as well as hematologic changes after G-CSF application are generally well tolerated and do not pose serious risks for most healthy donors. While serious acute toxicities have been reported, they are rare. Based on limited long-term data from healthy donors who received filgrastim, no long-term risks for development of leukemia, lymphoma or other blood diseases have been found (11,12). The only statistically increased cancer incidence was for melanoma, the significance of which is unclear. Reports of donor follow-up of blood cell counts, mostly within 5 years, find a sustained decrease from baseline in certain PB counts—particularly neutrophils—throughout the follow-up period, and this is especially pronounced in ‘poor mobilizers’ (12). This data perhaps hints at impaired hematopoiesis following PBSC donation, but even donors with normalized blood counts could harbor compromised HSC that might only be revealed through a stress event such as myelotoxic therapy, severe infection, or aging. The results of Bernitz and colleagues may suggest a need for longer-term donor follow-up studies to fully assess risks that may not be currently understood.
When considering surface area conversion of G-CSF dose from man to mouse (13) the model used by the Moore laboratory utilized a considerably higher dose than the 10 µg/kg/day typically used in humans for clinical mobilization. The mouse equivalent would be 125 µg/kg/day (14), while the current study injected 312.5 µg/kg/day for mobilization (reported as 6.25µg/day; assuming an approximate mouse weight of 20 g). Thus, at doses of G-CSF used clinically, severe depletion of donor LTR-HSC may not be a concern. Further support for this comes from clinical studies in which grafts derived from the BM upon G-CSF mobilization, or “G-CSF primed” BM, can engraft with similar outcomes to mobilized PB or untreated BM (15,16). Thus, residual donor BM may not be quite as functionally compromised after clinical G-CSF mobilization as that of this model.
It is widely acknowledged that G-CSF mobilized PBSC restore white blood cell counts, particularly absolute neutrophil counts, faster than BM cells, likely because there are more LTR-HSC in the G-CSF mobilized graft. The current paper brings out some interesting points in this regard. First, quiescent HSC with high regenerative potential were preferentially mobilized. This helps explain PBSC graft potency and likely contributes significantly to PBSC transplant success. At the same time, the authors have confirmed an earlier observation by our group (4) of a competitive defect in G-CSF mobilized PB compared to unmanipulated BM, and have further elucidated in elegant fashion that this defect is independent of HSC divisional history. In our hands, comparison of G-CSF mobilized mouse PB MNC to unmanipulated BM over a 4:1 to 1:1 dilution range indicates that the G-CSF mobilized graft contains approximately one third the competitive repopulating unit (CRU) potential of BM. Here, the authors performed a direct competitive transplant with equal numbers of LSK CD48– cells purified from each source, normalized for proliferative history by label retention, and found that BM cells still outcompeted PB cells at a ratio of 4:1 in primary and secondary transplants. Thus, it appears that mobilized HSC may indeed be functionally less potent, and due to something other than proliferation. In any case, it is likely that the increased engraftment of G-CSF mobilized PB seen clinically is highly dependent upon the increased numbers of LTR-HSC transplanted.
The reason behind the decreased potency of mobilized HSC is an intriguing question that remains to be explained. A good start would be to determine whether other mobilizing agents such as cyclophosphamide, GRO-beta, or AMD3100, which have been shown to work through different mechanisms (4,17), would also yield HSC with reduced function. If this phenomenon is not specific to G-CSF, then it may be a function of the change in environment. Cells of the BM niche are intricately involved in the preservation of stem cell properties (18) and exposure to increased oxygen tension is known to negatively impact HSC when removed from the hypoxic BM environment (19,20). Ultimately, if the precise mobilization-induced deficiency can be elucidated and corrected by additional treatment or altered protocols, this could potentially optimize the PBSC transplant process to achieve higher levels of success for patients and donors alike.
Furthermore, since we see here that HSC can have differential potency despite an identical proliferation history, the initial observation of this paper could be taken a step further to determine whether this G-CSF-induced defect is long-term or short-term; is this effect permanent or reversible? Recall that after the doxycycline chase, G-CSF treatment or control, and re-equilibration, equivalent numbers of LR-HSC were found in the BM. The LTR-HSC did not divide, but are they just as functional? It would be interesting to take the BM cells at this point and compare repopulation efficiency. The answer to this question could have bearing on both transplant donors and recipients regarding long-term effects of G-CSF on LTR-HSC.
Overall, is G-CSF positive or negative for stem cell function? We have seen here that G-CSF does not induce proliferation of the all-important LTR-HSC, contributing to their ultimate exhaustion as previously thought. However, G-CSF may leave donor BM more depleted of LTR-HSC than previously thought, potentially calling for longer-term donor follow-up studies. In addition, G-CSF mobilized HSC have lower repopulating potential per cell than untreated BM HSC, but can be more easily collected and in greater numbers. Ultimately, patient outcome is paramount and there are currently no significant survival differences between the two methods of HSC collection (2,3). The work in the Moore laboratory has provided important new insights towards understanding and improving the possibilities for HSC transplantation.
Acknowledgments
Funding: This work was supported by NIH grants HL096305, CA182947 and AG046246 to LM Pelus. AM Patterson is supported by NIH T32 training grant HL007910.
Footnote
Provenance and Peer Review: This article was commissioned and reviewed by Section Editor Peipei Xu, MD [Section Editor (Hematological Malignancies), Department of Hematology, The Affiliated Drum Tower Hospital of Nanjing University Medical School, Nanjing, China].
Conflicts of Interest: Both authors have completed the ICMJE uniform disclosure form (available at http://dx.doi.org/10.21037/aob.2017.06.02). The authors have no conflicts of interest to declare.
Ethical Statement: The authors are accountable for all aspects of the work in ensuring that questions related to the accuracy or integrity of any part of the work are appropriately investigated and resolved.
Open Access Statement: This is an Open Access article distributed in accordance with the Creative Commons Attribution-NonCommercial-NoDerivs 4.0 International License (CC BY-NC-ND 4.0), which permits the non-commercial replication and distribution of the article with the strict proviso that no changes or edits are made and the original work is properly cited (including links to both the formal publication through the relevant DOI and the license). See: https://creativecommons.org/licenses/by-nc-nd/4.0/.
References
- Bernitz JM, Daniel MG, Fstkchyan YS, et al. Granulocyte colony-stimulating factor mobilizes dormant hematopoietic stem cells without proliferation in mice. Blood 2017;129:1901-12. [Crossref] [PubMed]
- Anasetti C, Logan BR, Lee SJ, et al. Peripheral-blood stem cells versus bone marrow from unrelated donors. N Engl J Med 2012;367:1487-96. [Crossref] [PubMed]
- Holtick U, Albrecht M, Chemnitz JM, et al. Comparison of bone marrow versus peripheral blood allogeneic hematopoietic stem cell transplantation for hematological malignancies in adults - a systematic review and meta-analysis. Crit Rev Oncol Hematol 2015;94:179-88. [Crossref] [PubMed]
- Fukuda S, Bian H, King AG, et al. The chemokine GRObeta mobilizes early hematopoietic stem cells characterized by enhanced homing and engraftment. Blood 2007;110:860-9. [Crossref] [PubMed]
- Morrison SJ, Wright DE, Weissman IL. Cyclophosphamide/granulocyte colony-stimulating factor induces hematopoietic stem cells to proliferate prior to mobilization. Proc Natl Acad Sci U S A 1997;94:1908-13. [Crossref] [PubMed]
- Walter D, Lier A, Geiselhart A, et al. Exit from dormancy provokes DNA-damage-induced attrition in haematopoietic stem cells. Nature 2015;520:549-52. [Crossref] [PubMed]
- Wright DE, Cheshier SH, Wagers AJ, et al. Cyclophosphamide/granulocyte colony-stimulating factor causes selective mobilization of bone marrow hematopoietic stem cells into the blood after M phase of the cell cycle. Blood 2001;97:2278-85. [Crossref] [PubMed]
- Foudi A, Hochedlinger K, Van Buren D, et al. Analysis of histone 2B-GFP retention reveals slowly cycling hematopoietic stem cells. Nat Biotechnol 2009;27:84-90. [Crossref] [PubMed]
- Qiu J, Papatsenko D, Niu X, et al. Divisional history and hematopoietic stem cell function during homeostasis. Stem Cell Reports 2014;2:473-90. [Crossref] [PubMed]
- Wilson A, Laurenti E, Oser G, et al. Hematopoietic stem cells reversibly switch from dormancy to self-renewal during homeostasis and repair. Cell 2008;135:1118-29. [Crossref] [PubMed]
- Confer DL, Miller JP. Long-term safety of filgrastim (rhG-CSF) administration. Br J Haematol 2007;137:77-8. [Crossref] [PubMed]
- Hölig K. G-CSF in Healthy Allogeneic Stem Cell Donors. Transfus Med Hemother 2013;40:225-35. [Crossref] [PubMed]
- Reagan-Shaw S, Nihal M, Ahmad N. Dose translation from animal to human studies revisited. FASEB J 2008;22:659-61. [Crossref] [PubMed]
- Hoggatt J, Tate TA, Pelus LM. Hematopoietic stem and progenitor cell mobilization in mice. Methods Mol Biol 2014;1185:43-64. [Crossref] [PubMed]
- Arcese W, De Angelis G, Cerretti R. Granulocyte-mobilized bone marrow. Curr Opin Hematol 2012;19:448-53. [Crossref] [PubMed]
- Elfenbein GJ, Sackstein R. Primed marrow for autologous and allogeneic transplantation: a review comparing primed marrow to mobilized blood and steady-state marrow. Exp Hematol 2004;32:327-39. [Crossref] [PubMed]
- Winkler IG, Pettit AR, Raggatt LJ, et al. Hematopoietic stem cell mobilizing agents G-CSF, cyclophosphamide or AMD3100 have distinct mechanisms of action on bone marrow HSC niches and bone formation. Leukemia 2012;26:1594-601. [Crossref] [PubMed]
- Anthony BA, Link DC. Regulation of hematopoietic stem cells by bone marrow stromal cells. Trends Immunol 2014;35:32-7. [Crossref] [PubMed]
- Mantel CR, O'Leary HA, Chitteti BR, et al. Enhancing Hematopoietic Stem Cell Transplantation Efficacy by Mitigating Oxygen Shock. Cell 2015;161:1553-65. [Crossref] [PubMed]
- Spencer JA, Ferraro F, Roussakis E, et al. Direct measurement of local oxygen concentration in the bone marrow of live animals. Nature 2014;508:269-73. [Crossref] [PubMed]
Cite this article as: Patterson AM, Pelus LM. G-CSF in stem cell mobilization: new insights, new questions. Ann Blood 2017;2:10.