Predictive Model of variable laboratory findings calculation and monitoring during plasmapheresis procedures
Highlight box
Key findings
• Theoretical model was developed to show the beneficial possibilities to be installed in the software for continuously available laboratory monitoring. Further development would work on actual donor data with additional features for microelements.
What is known and what is new?
• Interactive model for lipoproteins fluctuation during plasmapheresis procedure can be accessed using active link—http://lpmc.labdata.lt/.
• This manuscript concludes possible plasmapheresis impact on lipoproteins and discusses its mechanisms.
What is the implication, and what should change now?
• This model may increase people’s interest in donation procedure. Following the published results, couple of positive triggers, motivating them to donate, were found to be an awareness of free health checkup and expectations of physical benefits for the body (Tebabal et al. 2023). The Predictive Model emphasizes possible insights for cholesterol metabolism won’t be limited to with further proposals on proteins, microelements and enzymes monitoring.
Introduction
Plasmapheresis is a therapeutic intervention that separates whole blood cellular components from plasma using centrifugation or filtration by semipermeable membranes. Separated cells then are reinfused into the bloodstream with autologous plasma or another replacement solution (1). There are significant benefits for recipients of donated plasma, but potential benefits for donors are poorly described in literature. Currently the main scientific focus for donors is—to determine how many milliliters can be drawn and how often plasma can be donated to guarantee no harm to the donor (2). A full landscape of the plasma donation process and evaluation of homeostasis during procedures could clarify the possible physiological benefits of plasmapheresis to the plasma donor.
We have developed the template of Predictive Model, based on published results by Rosa-Bray et al. (3), and theoretically designed it to simplify analysis of the data that may be obtained continuously via plasmapheresis procedures. A study by Rosa-Bray and colleagues described findings of reduced levels of low-density lipoprotein (LDL) and increased yield of high-density lipoprotein (HDL) after numerous of plasmapheresis. Altered lipid profile (elevated LDL, decreased HDL levels, hypertriglyceridemia) is associated with many pathological health conditions such as diabetes mellitus, metabolic syndrome, atherosclerosis, and cardiovascular diseases (4-6). While researchers search for the new treatment modalities for such patients, any insights that could help to improve one’s lipid profile are valuable. The understanding of possible mechanisms behind previously described plasmapheresis induced alterations in plasma lipid concentrations may contribute to understanding the complex multifactorial pathogenesis of metabolic diseases related with dyslipidemia leading to more efficient options of treatment. The mechanisms responsible for such changes are not defined and required further studies.
Our aim is to explore the feasible options that may be applied to monitor the concentration of lipoproteins in blood samples. Predicting cholesterol metabolism changes during plasmapheresis procedures emphasizes the attractive value of plasmapheresis process for donors. Following the published results, couple of positive triggers, motivating them to donate, were found to be an awareness of free health checkup and expectations of physical benefits for the body (7). The model so far serves only demonstrative purpose and needs further development based on a large scope research.
Background
General lipoprotein chemistry and functions
Lipoproteins are complex sphere-like heterogenic particles produced by liver and intestines that mainly function to facilitate dietary or endogenous lipids transport in blood circulation (8-10). There are six classes of lipoproteins: chylomicrons, very LDL (VLDL), intermediate density lipoprotein (IDL), LDL, high density lipoprotein (HDL), and lipoprotein (a) [Lp (a)]. The most numerous particles are LDL, and they can comprise >90% of plasma apoB-containing lipoproteins (11,12).
The exogenous lipoprotein pathway starts in enterocytes with chylomicron synthesis from dietary lipids which are mostly triglycerides. Newly synthesized chylomicrons contain apoB-48 and flow with lymph into the blood. Capillary endothelial lipoprotein lipase causes triglycerides hydrolysis. Released free fatty acids (FFAs) can be utilized by muscle cells or stored in adipocytes (13). Chylomicron remnants have high cholesterol content and through apo-E binding are taken up by hepatocytes from circulation and recycled (10).
The endogenous lipoprotein pathway starts in the liver with the formation of VLDL mainly through de novo lipogenesis. Within circulation, the fate of VLDLs is similar to chylomicrons as they are hydrolyzed by endothelial lipoprotein lipase (14). Following triglyceride depletion, IDLs are formed and can be utilized by the liver or converted to LDLs by further metabolism (15). LDLs increase the amount of intracellular cholesterol (16).
HDLs are responsible for reverse cholesterol transport and acquire excessive cholesterol and other compounds from peripheral tissues and facilitate transport to the liver directly or indirectly by transferring cholesterol to VLDL or LDL (17). HDLs have antioxidative properties and reduce cholesterol levels in subendothelial space of blood vessels (18,19).
Rationale and knowledge gap
The demand for plasma-derived medicinal products has increased by 7–8% per year. It was estimated that Europe needs 2 million extra donors of blood and plasma (20). To achieve this goal plasmapheresis procedures should become more donor orientated ensuring better donor care and safety, researching or even adapting the procedure for beneficial aspects to the donor. A study by Rosa-Bray and colleagues (3) found that continuous plasmapheresis procedures may lower cholesterol concentrations in patients with hypercholesterolemia. This study analyzed articles that may explain the related physiology. Defining the mechanisms of how plasmapheresis impacts blood lipids and homeostasis may result in a range of blood tests that may be important to monitor during continuous plasmapheresis procedures. This study focused on creating a prototype for such a program that could be installed for continuously available laboratory monitoring during plasmapheresis, track changes in donors blood lipids, proteins or minerals concentration. This program would ensure donors’ safety and may detect and analyze favorable changes in blood chemistry profile such as lowered cholesterol.
Objective
To develop an Interactive Model investigating the body’s response to plasmapheresis procedures to be installed in the software for continuously available laboratory monitoring.
Methods
Literature review was performed using National Center for Biotechnology Information (NCBI) Literature Resources based on thematic analysis with keywords: “lipoproteins in plasma”, “cholesterol chemistry”, “plasma donors and lipoproteins”, “plasmapheresis and lipids”. Filters for full text, original study, review and metanalysis, not older than 10 years were selected; 76 available sources were found and 58 were left for final revision during the literature search from 5 March to 10 June 2024. The main reason for exclusion was secondary citations or references of the primary articles.
We used Microsoft Excel program to perform theoretical calculations, based on the data published by Rosa-Bray and team (3). Based on the data—663 donors’ samples were tested (Rosa-Bray, 2013). The model first calculates the approximate total blood volume of the donor according to physical parameters. Donated plasma volume depends on donor’s weight and proportionally to removed plasma the model predicts immediate decrease of cholesterol fractions concentration in blood after each donation procedure. Following the sudden drop in cholesterol concentration homeostasis reacts by increasing cholesterol synthesis. The tendencies for changes from baseline cholesterol during continuous plasmapheresis procedures were outlined in Results section Table 1. To create a user-friendly model interface, custom programming with web technologies was used (e.g., HTML, CSS, Javascript). The following libraries amCharts, Google Charts were used to create program prototype. All programming was done with Visual Studio Code software.
Table 1
Variables | Hypercholesterolemia status (total cholesterol ≥5.18 mmol/L) | Normal cholesterol status (total cholesterol <5.18 mmol/L) |
---|---|---|
Male donors | ||
Total cholesterol | ↓ 0.3* | ↑ 0.16* |
LDL cholesterol | ↓ 0.27‡ | ↑ 0.16* |
HDL cholesterol | ↑ 0.06* | ↓ 0.13‡ |
Female donors | ||
Total cholesterol | ↓ 0.53* | ↑ 0.08* |
LDL cholesterol | ↓ 0.4* | ↑ 0.1* |
HDL cholesterol | ↑ 0.03* | ↓ 0.17‡ |
Adapted from Rosa-Bray et al., 2013 (3). *, P<0.01; ‡, P<0.05. LDL, low-density lipoprotein; HDL, high-density lipoprotein.
Statistical analysis
No statistical analysis was conducted as theoretical computing model was based on data gathered by Rosa-Bray et al. study (3).
Results
“Predictive Model of variable laboratory findings calculation and monitoring during plasmapheresis procedures” (initially based on lipid clinical profile) can be accessed using active link—http://lpmc.labdata.lt/. The model represents only approximate and demonstrative values, serving as prototype for the routine solutions based on lipoproteinemia levels monitor. The main result of this study is to present a visual template of Predictive Model. It serves as a theoretical basis for a more dynamic and expanded version that can be created as a result of evidence-based research to be suitable for plasmapheresis systems software.
This novel “Predictive Model of variable laboratory findings calculation and monitoring during plasmapheresis procedures” starts with a request to fill in descriptive donor data: gender, age, height, weight, hypercholesterolemia status (Figure 1). This information can be used for statistical analysis. Gender, weight, and height are also important for calculation of approximate blood volume to obtain according to Nadler’s equation:
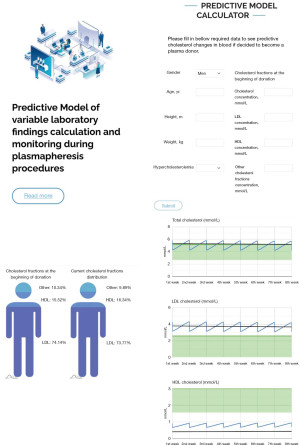
where H, height (m); W, weight (kg).
Donated plasma volume depends on donor’s weight: 690 mL (<90 kg), 825 mL (90–113 kg), 880 mL (>113 kg).
Beside descriptive donor information, the model has the input function of initial actual total, LDL and HDL cholesterol concentrations. According to the data, the model predicts and designs graphical illustrations of cholesterol and its fraction distribution at the beginning and at the last donation event to compare the changes in blood. There are three diagrams at the bottom of the program window showing total, LDL, HDL cholesterol concentration fluctuations during plasma donation period.
After plasma donation all cholesterol fractions levels in blood drop down because of lipoprotein removal, which is dependent on donated plasma volume. Further cholesterol metabolism rate depends on various factors such as days between donation, gender and possible hypercholesterolemia status. For demonstration purpose we chose 7 days interval between donations and designed two different possible scenarios with fluctuations patterns of fractions depending on the status of cholesterol metabolism:
- In the case of hypercholesterolemia—total and LDL cholesterol concentration tends to decrease while HDL increases during plasma donation period.
- In the case of normal cholesterol values—total and LDL cholesterol have a very slight tendency to increase and HDL decrease.
Criteria for the tendencies were defined according to Rosa-Bray and team (3) research on predictive effect of plasmapheresis on change from baseline to final serial procedure in total, LDL, HDL cholesterol.
Discussion
Key findings
The Predictive Model of possible lipoproteinemia fluctuations during the plasma donation period was hypothetically designed according to the published data by Rosa-Bray and colleagues (3). The Model was developed to highlight the potential benefits, for instance, for the software to be installed during plasmapheresis combined with rapid laboratory diagnostics further development may demonstrate an attractive approach of practical application.
Strengths and limitations
Additional features for microelements, such as zinc (Zn), copper (Cu), iron (Fe) also have capacity to be discussed, based on recently published studies by Knez with colleagues (21). They found a possible link for fatty acid desaturation to the Zn intake and metabolism. Also, similar evaluation of proteins: albumin, globulin production, concentration alterations during plasmapheresis may be beneficial (22). Highlighting the mechanisms related to plasma substances transport (23) and tracking details of extended laboratory investigation with addition of other assays for further analysis may take place in developing our Predictive Model prototype to become useful in practice.
Donated plasma volume depends on donors’ weight (24). Our theoretical model so far has two different possible cholesterol and its fractions concentration fluctuations patterns depending on cholesterol metabolism status. In case of hypercholesterolemia, total and LDL cholesterol concentrations tend to decrease while HDL increases during plasma donation period. However, if a donor has normal cholesterol values, the concentration changes are more difficult to predict, total and LDL cholesterol may even have a very slight tendency to increase because of disturbed normal cholesterol metabolism due to variable reasons (25).
It would be interesting to investigate how cholesterol metabolism adapts to external influences of plasmapheresis. Specifically, to understand how abnormal cholesterol values may drop in reference range after donation or how the percentage distribution of cholesterol fractions shifts in each donor case depending on medical history and metabolic status (26).
Assuming plasma donors are healthy people, and their nutritional status is adequate according to the dieticians’ guidelines (27), no additional exemptions are required for estimated calculation of lipoproteins at this point.
Comparison with similar researches
We were not able to find a similar model creation or description in the literature search.
Explanations of findings
Discussion could arise on microelements during plasmapheresis, as limited studies have been currently performed. Exceptions are therapeutical plasma exchange benefits for patients with pediatric acute liver failure, accepted as one of the treatment methods (28) or efficacy of lipoprotein apheresis treating homozygous familial hypercholesterolemia with the benefits of reducing LDL and lowering cardiovascular risk (29) and lipopheresis positive effect for dementia treatment (30) or more clinical application examples of similar therapeutical plasma exchange (31) resulting effect on cholesterol fractions outcomes. During the process of plasmapheresis, changes in proteins and salt concentration could arise, as well as dehydration; especially due to insufficient food and water intake (32). Recent study from 255 United States plasma centers found the most relevant reasons for plasma donor deferral, besides high blood pressure and pulse rate, was stated low protein and low hematocrit levels. This occurs predominantly 80% for females (33).
It is worth emphasizing the possible plasmapheresis general impact on lipoproteins, speculating on pre-made cholesterol consumption. Familial hypercholesterolemia is an inherited lipoprotein metabolism disorder resulting in an increased total and LDL cholesterol and one of the possible treatments is LDL apheresis. It has been shown to be a very beneficial treatment option that reduces cholesterol levels and inhibits progression of atherosclerotic lesions (34). As mentioned in the introduction, plasmapheresis for plasma donation purposes may also lower cholesterol levels in donors with elevated baseline cholesterol levels (3). It is possible that patients with high baseline total and LDL cholesterol may have subclinical cholesterol deposits in vascular and peripheral tissues (35). During plasmapheresis human body homeostasis experiences a sudden drop in cholesterol concentration because lipoproteins are removed from circulation with donated plasma. Cholesterol synthesis de novo is energetically expensive and requires additional resources, so it supplements exogenous supply based on demand (36). The liver reacts to this increased cellular demand for cholesterol after plasmapheresis and uses the pre-made cholesterol from the deposits in peripheral tissues. This mechanism may explain elevated HDL levels in donors experiencing continuous plasmapheresis (3). Similarly, LDL alterations during apheresis for donors with hypercholesterolemia may also be slightly protective against atherosclerotic plaque formation.
Another known and widely discussed mechanism, the “shuttle and sink”, cause physiological effect of substances in the body (37). Albumin is the main human body protein with many biological functions. It maintains colloidal osmotic pressure and acts as a transport protein for FFAs, other lipids, and various endo-/exogenous substances. Albumin also binds essential metal ions such as Zn to allow their systemic distribution (38). Another function arises when it comes to albumin mediated transport—role in the diffusion pathway for cholesterol. Cholesterol molecules flux between extracellular particles and various cells and there are numerous publications addressing different mechanisms (37,39). Considering the plasmapheresis principle, efflux via aqueous diffusion pathways suggest more insights on possible free cholesterol motion that may occur during plasmapheresis. Albumin has its role obviously. Cholesterol molecules diffuse from cells into aqueous medium to integrate with recipient particles [either lipoproteins or red blood cells (RBCs)] which act as “sinks”. Because cholesterol molecules are poorly soluble in aqueous medium, albumin works as a “shuttle” and enhances this diffusion making it more efficient because this way cholesterol easily distributes among lipoproteins, various cells and different medium compartments (37). It is possible, that via mediating this albumin “shuttle”, RBCs act as a free cholesterol “sink” for the donor during the plasmapheresis procedure as well, because of the plasma dilution effect. New data suggests that the fractional efflux of cholesterol molecules in plasma is greater with the combination of albumin and RBC model. Albumin transports cholesterol molecules by binding cholesterol and increasing the concentration of the sterols in the aqueous phase, and additionally albumin can enhance movement of free cholesterol between cells. It is possible, that while donors’ plasma is removed and replaced with albumin solution mainly, the “shuttle and sink” mechanism takes place to alter cholesterol concentrations due to RBC and albumin concentrations in blood stream is rapidly increasing.
FFAs and Zn relationship via binding to albumin will be explored. Zn is an essential trace mineral which is widely distributed within various cells. It has significant activity for multiple metabolic processes such as gene expression, immunity modulation, enzyme activity regulation (40,41). Approximately 75–90% of plasma Zn is bound to albumin, so even small changes in albumin’s capacity to bind Zn may result in a significant metabolic shift. An interesting relationship between FFAs concentration and Zn distribution via binding to albumin is worth to be discussed (42). FFAs circulate predominantly bound to albumin in the bloodstream (43). FFAs provide energy as an alternative source to glucose especially under conditions requiring higher energy consumption. However, continuous excessive FFAs in blood is a risk factor, leading to the lipid deposition in liver and peripheral tissues, insulin resistance, and vascular and cardiac dysfunction (43,44). Albumin can bind excessive FFAs in cases of physiological (fasting, exercising) or pathological (insulin resistance, obesity) conditions. However, FFAs binding to albumin can significantly change the distribution of Zn in plasma through allosteric regulation. The albumin Zn binding site is disrupted when an FFA molecule (containing more than 10 carbon atoms) binds to albumin thus releasing bound Zn through a ‘spring-lock’ mechanism. The decrease in albumin Zn binding capacity shifts the distribution of Zn causing Zn efflux from plasma to tissues. This effect is more pronounced with higher FFAs amounts in blood (42). Consequently, decreased plasma Zn concentration may cause a negative impact on health. Plasmapheresis has been proven to effectively reduce excess triglycerides levels in circulation and a single session of therapeutic plasmapheresis can reduce triglycerides by an average of 70% (45). We hypothesize that plasmapheresis for donation purpose may also lower triglycerides and FFAs concentration thus decreasing albumin saturation with FFAs leading to an increased albumin Zn binding capacity and favorable health outcomes.
Implications and actions needed
Based on the literature and gathered data, this novel approach to study the impact of plasmapheresis on lipoprotein concentrations should be considered for future clinical investigation. There are more functions of lipoproteins besides transporting lipids in the blood stream. Looking more broadly, they can bind lipophilic molecules such as bacterial lipopolysaccharides (LPS, endotoxin) from gram-negative bacteria and lipoteichoic acid from gram-positive bacteria (10). Mycotoxins are also lipophilic and can be incorporated in the surface of lipoprotein particles with sequestration by cholesterol (46). Lipophilic toxins are slowly excreted and must be metabolized into more hydrophilic metabolites through detoxification systems (47). Circulating lipoprotein bound toxins are less aggressive, but with prolonged circulation they can still interact with cells and various surface structures thus inducing pathological processes (10,48). LPS and mycotoxins can cause post transcriptional and post translational modifications, disturb cholesterol and lipoprotein metabolism, impact membrane apo E/A interactions leading to hypercholesterolemia, result in low HDL levels, and systemic inflammation, lipid peroxidation, macrophage activation (46,48-52). Industrial lipophilic xenobiotics may have an even more volatile nature as they can upregulate autoimmune diseases related gene expression in susceptible patients and enhance inflammatory response through binding with aryl hydrocarbons, nucleic acids, proteins thus forming neoantigens, deregulating epigenetic mechanisms, disrupting immune barrier systems and depleting antioxidants such as glutathione (53).
Because of genetic variants, some detoxification pathways tend to be slower resulting in accumulation of corresponding lipophilic toxins (54,55). It may be possible that during each session of plasmapheresis a portion of circulating lipoproteins are being removed alongside with small amounts of toxic lipophilic substances especially those that tend to accumulate because of genetically determined impairment of some detoxification pathways. This may ease liver burden if the donor has been exposed to natural or synthetic toxic substances that are soluble in lipids. The possible plasmapheresis value for such affected clinical patients should be further explored by studying multiple plasmapheresis effect for plasma donors.
Conclusions
The theoretical model of cholesterol concentration changes monitor during plasmapheresis within eight weeks serves as example for prediction and justifies beginning of more extensive study, involving expanded laboratory analysis on proteins, microelements, enzymes, etc.
The application in practice may increase people’s interest in donation procedure. Different options for presentation of results in simple, attractive design are possible and further investigations should be conducted.
Acknowledgments
We appreciated to Joint Stock Company LabDate’s IT solutions team for excellent fulfillment of our ideas while designing the Computing Model.
Funding: None.
Footnote
Peer Review File: Available at https://aob.amegroups.com/article/view/10.21037/aob-24-16/prf
Conflicts of Interest: All authors have completed the ICMJE uniform disclosure form (available at https://aob.amegroups.com/article/view/10.21037/aob-24-16/coif). R.S., N.R. and M.A. reported this manuscript was prepared under the Collaborative Research Study Project “Predictive Model of variable laboratory findings calculation and monitoring during plasmapheresis procedure”. The other authors have no conflicts of interest to declare.
Ethical Statement: The authors are accountable for all aspects of the work in ensuring that questions related to the accuracy or integrity of any part of the work are appropriately investigated and resolved.
Open Access Statement: This is an Open Access article distributed in accordance with the Creative Commons Attribution-NonCommercial-NoDerivs 4.0 International License (CC BY-NC-ND 4.0), which permits the non-commercial replication and distribution of the article with the strict proviso that no changes or edits are made and the original work is properly cited (including links to both the formal publication through the relevant DOI and the license). See: https://creativecommons.org/licenses/by-nc-nd/4.0/.
References
- Sergent SR, Ashurst JV. Plasmapheresis (Internet). In: StatPearls. Treasure Island (FL): StatPearls Publishing. 2022 (Updated 2022 July 12; cited 2022 December 7). Available online: https://www.ncbi.nlm.nih.gov/books/NBK560566/
- Hoad VC, Castrén J, Norda R, et al. A donor safety evidence literature review of the short- and long-term effects of plasmapheresis. Vox Sang 2024;119:94-101. [Crossref] [PubMed]
- Rosa-Bray M, Wisdom C, Wada S, et al. Prospective multicentre study of the effect of voluntary plasmapheresis on plasma cholesterol levels in donors. Vox Sang 2013;105:108-15. [Crossref] [PubMed]
- Jialal I, Singh G. Management of diabetic dyslipidemia: An update. World J Diabetes 2019;10:280-90. [Crossref] [PubMed]
- Paredes S, Fonseca L, Ribeiro L, et al. Novel and traditional lipid profiles in Metabolic Syndrome reveal a high atherogenicity. Sci Rep 2019;9:11792. [Crossref] [PubMed]
- Hedayatnia M, Asadi Z, Zare-Feyzabadi R, et al. Dyslipidemia and cardiovascular disease risk among the MASHAD study population. Lipids Health Dis 2020;19:42. [Crossref] [PubMed]
- Tebabal B, Anagaw TF, Adamu A, et al. Factors Influencing Blood Donation Practice Among Health Care Providers of Public Hospitals in Bahir Dar City, North West Ethiopia: A Case Control Study. J Blood Med 2023;14:487-98. [Crossref] [PubMed]
- Busatto S, Walker SA, Grayson W, et al. Lipoprotein-based drug delivery. Adv Drug Deliv Rev 2020;159:377-90. [Crossref] [PubMed]
- Visseren FLJ, Mach F, Smulders YM, et al. 2021 ESC Guidelines on cardiovascular disease prevention in clinical practice. Eur Heart J 2021;42:3227-337. [Crossref] [PubMed]
- Feingold KR. Lipid and Lipoprotein Metabolism. Endocrinol Metab Clin North Am 2022;51:437-58. [Crossref] [PubMed]
- Borén J, Williams KJ. The central role of arterial retention of cholesterol-rich apolipoprotein-B-containing lipoproteins in the pathogenesis of atherosclerosis: a triumph of simplicity. Curr Opin Lipidol 2016;27:473-83. [Crossref] [PubMed]
- Ference BA, Graham I, Tokgozoglu L, et al. Impact of Lipids on Cardiovascular Health: JACC Health Promotion Series. J Am Coll Cardiol 2018;72:1141-56. [Crossref] [PubMed]
- Lent-Schochet D, Jialal I. Biochemistry, Lipoprotein Metabolism. In: StatPearls. Treasure Island (FL): StatPearls Publishing; January 16, 2023.
- Salih KJ. The major pathways of lipids (triglyceride and cholesterol) and lipoprotein metabolism. Zanco Journal of Pure and Applied Sciences 2021;33:61-72.
- Ivanova EA, Myasoedova VA, Melnichenko AA, et al. Small Dense Low-Density Lipoprotein as Biomarker for Atherosclerotic Diseases. Oxid Med Cell Longev 2017;2017:1273042. [Crossref] [PubMed]
- Luo J, Yang H, Song BL. Mechanisms and regulation of cholesterol homeostasis. Nat Rev Mol Cell Biol 2020;21:225-45. [Crossref] [PubMed]
- Kosmas CE, Martinez I, Sourlas A, et al. High-density lipoprotein (HDL) functionality and its relevance to atherosclerotic cardiovascular disease. Drugs Context 2018;7:212525. [Crossref] [PubMed]
- Eckardstein A, Kardassis D. High Density Lipoproteins. From Biological Understanding to Clinical Exploitation. 2015; Vol. 224.
- Jomard A, Osto E. High Density Lipoproteins: Metabolism, Function, and Therapeutic Potential. Front Cardiovasc Med 2020;7:39. [Crossref] [PubMed]
- Soto-Vega E, Rivadeneyra-Espinoza L, Cuan-Baltazar Y, et al. The “sticky platelet syndrome”: thirty years after its identification in Mexico. Ann Blood 2024;9:17. [Crossref]
- Knez M, Pantovic A, Zekovic M, et al. Is There a Link between Zinc Intake and Status with Plasma Fatty Acid Profile and Desaturase Activities in Dyslipidemic Subjects? Nutrients 2019;12:93. [Crossref] [PubMed]
- van de Wouw J, Joles JA. Albumin is an interface between blood plasma and cell membrane, and not just a sponge. Clin Kidney J 2022;15:624-34. [Crossref] [PubMed]
- Pizzagalli MD, Bensimon A, Superti-Furga G. A guide to plasma membrane solute carrier proteins. FEBS J 2021;288:2784-835. [Crossref] [PubMed]
- Jerrard GA, Liu J, Case RC, et al. Implications of weight and body mass index for plasma donation and health. ISRN Hematol 2012;2012:937585. [Crossref] [PubMed]
- Platt FM, Wassif C, Colaco A, et al. Disorders of cholesterol metabolism and their unanticipated convergent mechanisms of disease. Annu Rev Genomics Hum Genet 2014;15:173-94. [Crossref] [PubMed]
- Infante RE, Radhakrishnan A. Continuous transport of a small fraction of plasma membrane cholesterol to endoplasmic reticulum regulates total cellular cholesterol. Elife 2017;6:e25466. [Crossref] [PubMed]
- Berger MM, Shenkin A, Schweinlin A, et al. ESPEN micronutrient guideline. Clin Nutr 2022;41:1357-424. [Crossref] [PubMed]
- Alexander EC, Deep A. Therapeutic plasma exchange in children with acute liver failure (ALF): is it time for incorporation into the ALF armamentarium? Pediatr Nephrol 2022;37:1775-88. [Crossref] [PubMed]
- Lischka J, Arbeiter K, de Gier C, et al. Vascular access for lipid apheresis: a challenge in young children with homozygous familial hypercholesterolemia. BMC Pediatr 2022;22:131. [Crossref] [PubMed]
- Orehek AJ. Dementia Improvement after Plasma Exchange for Familial Hypercholesterolemia. Case Rep Neurol Med 2016;2016:6121878. [Crossref] [PubMed]
- Filipov JJ, Zlatkov BK, Dimitrov EP. Plasma Exchange in Clinical Practice (Internet). Plasma Medicine - Concepts and Clinical Applications. InTech; 2018. Available online:
10.5772/intechopen.76094 10.5772/intechopen.76094 - Liu B, Dong D, Wang Z, et al. Analysis of influencing factors of serum total protein and serum calcium content in plasma donors. PeerJ 2022;10:e14474. [Crossref] [PubMed]
- Schmidt AE, MacKercher J, Youngling B, et al. Source plasma deferral trends: A 3-year analysis of 255 centers in the United States. J Clin Apher 2022;37:31-9. [Crossref] [PubMed]
- Taylan C, Weber LT. An update on lipid apheresis for familial hypercholesterolemia. Pediatr Nephrol 2023;38:371-82. [Crossref] [PubMed]
- Gatto L, Prati F. Subclinical atherosclerosis: how and when to treat it? Eur Heart J Suppl 2020;22:E87-90. [Crossref] [PubMed]
- Brown AJ, Sharpe LJ. Chapter 11 – Cholesterol Synthesis. Biochemistry of Lipids, Lipoproteins and Membranes (Sixth Edition). Elsevier; 2016:327-58.
- Sankaranarayanan S, de la Llera-Moya M, Drazul-Schrader D, et al. Serum albumin acts as a shuttle to enhance cholesterol efflux from cells. J Lipid Res 2013;54:671-6. [Crossref] [PubMed]
- Moman RN, Gupta N, Varacallo M. Physiology, Albumin. In: StatPearls. Treasure Island (FL): StatPearls Publishing; 2024.
- Kilsdonk EP, Yancey PG, Stoudt GW, et al. Cellular cholesterol efflux mediated by cyclodextrins. J Biol Chem 1995;270:17250-6. [Crossref] [PubMed]
- Li J, Cao D, Huang Y, et al. Zinc Intakes and Health Outcomes: An Umbrella Review. Front Nutr 2022;9:798078. [Crossref] [PubMed]
- Roohani N, Hurrell R, Kelishadi R, et al. Zinc and its importance for human health: An integrative review. J Res Med Sci 2013;18:144-57. [PubMed]
- Coverdale JPC, Khazaipoul S, Arya S, et al. Crosstalk between zinc and free fatty acids in plasma. Biochim Biophys Acta Mol Cell Biol Lipids 2019;1864:532-42. [Crossref] [PubMed]
- I S Sobczak A. A Blindauer C, J Stewart A. Changes in Plasma Free Fatty Acids Associated with Type-2 Diabetes. Nutrients 2019;11:2022. [Crossref] [PubMed]
- Henderson GC. Plasma Free Fatty Acid Concentration as a Modifiable Risk Factor for Metabolic Disease. Nutrients 2021;13:2590. [Crossref] [PubMed]
- Munoh Kenne F, Cimpeanu E, Al-Zakhari R, et al. Plasmapheresis Treatment of Hypertriglyceridemia-Induced Acute Pancreatitis: A Case Report. Cureus 2020;12:e8360. [Crossref] [PubMed]
- Martins IJ. Overnutrition Determines LPS Regulation of Mycotoxin Induced Neurotoxicity in Neurodegenerative Diseases. Int J Mol Sci 2015;16:29554-73. [Crossref] [PubMed]
- Chen G. Chapter 3 – Xenobiotic metabolism and disposition. An introduction to Interdisciplinary Toxicology. Elsevier; 2020:31-42.
- Malvandi AM, Shahba S, Mehrzad J, et al. Metabolic Disruption by Naturally Occurring Mycotoxins in Circulation: A Focus on Vascular and Bone Homeostasis Dysfunction. Front Nutr 2022;9:915681. [Crossref] [PubMed]
- Wang K, Liu S, Zhang X, et al. Toxic Effect of Mycotoxins on Cardiovascular System: A Topic Worthy of Further Study. Food Reviews International 2021;39:2203-11. [Crossref]
- Del Favero G, Hohenbichler J, Mayer RM, et al. Mycotoxin Altertoxin II Induces Lipid Peroxidation Connecting Mitochondrial Stress Response to NF-κB Inhibition in THP-1 Macrophages. Chem Res Toxicol 2020;33:492-504. [Crossref] [PubMed]
- Carvajal-Moreno M. Mycotoxins that Affect the Human Cardiovascular System. J Biomol Res Ther 2015;4:124.
- Ross MK, Borazjani A, Mangum LC, et al. Effects of toxicologically relevant xenobiotics and the lipid-derived electrophile 4-hydroxynonenal on macrophage cholesterol efflux: silencing carboxylesterase 1 has paradoxical effects on cholesterol uptake and efflux. Chem Res Toxicol 2014;27:1743-56. [Crossref] [PubMed]
- Kharrazian D. Exposure to Environmental Toxins and Autoimmune Conditions. Integr Med (Encinitas) 2021;20:20-4. [PubMed]
- Aronica L, Ordovas JM, Volkov A, et al. Genetic Biomarkers of Metabolic Detoxification for Personalized Lifestyle Medicine. Nutrients 2022;14:768. [Crossref] [PubMed]
- Zmrzljak UP, Rozman D. Circadian regulation of the hepatic endobiotic and xenobitoic detoxification pathways: the time matters. Chem Res Toxicol 2012;25:811-24. [Crossref] [PubMed]
Cite this article as: Jacike Z, Rajackaite E, Sepetiene R, Robbins N, Ali M. Predictive Model of variable laboratory findings calculation and monitoring during plasmapheresis procedures. Ann Blood 2024;9:24.