The history of thrombotic thrombocytopenic purpura research: a narrative review
Introduction
A 16-year-old girl presented with 1 week of fever, joint pain, and progressive upper extremity weakness to Beth Israel Hospital in New York City in 1924 (1,2). Eli Moschcowitz, a Hungarian-born internist, worked at that hospital at the time and published reports describing her clinical course. The patient was pale with scattered petechiae, with laboratory evaluation consistent with anemia. The patient developed renal failure early on in her clinical course. Her condition worsened. She went into a coma, and soon after died. Autopsy revealed “pale myocardium” and “hyaline” thrombotic occlusion of terminal arterioles and capillaries, consistent with a microvascular thrombotic component to the disease that ultimately decided the fate of this child. This was the first case report describing what eventually became known as Moschcowitz disease, which we now know as thrombotic thrombocytopenic purpura (TTP) (3-5).
The history of TTP exemplifies the best application of the scientific method. Hundreds of scientists and physicians, both sung and unsung, have come together through the generations to not only identify and describe this serious and deadly rare disease, but to render it a treatable illness. Any questions worth investing the time and energy to answer require open and curious minds to share their ideas, accept their mistakes, and amplify their successes. Here, we will describe some of the major discoveries in the past 100 years that have led us to our current understanding of the pathophysiology and optimal treatment paradigms for TTP. We present this article in accordance with the Narrative Review reporting checklist (available at https://aob.amegroups.org/article/view/10.21037/aob-23-46/rc).
Methods
We performed a literature search through PubMed from 1924 to 2023 using free text: “thrombotic thrombocytopenic purpura”, “Moschcowitz disease”, and “thrombotic microangiopathy” (Table 1). We found a total of 6,716, 17, and 4,637 peer-reviewed articles, respectively. Of these, the vast majority did not specifically outline the history of TTP, while we found 46 references sufficient for an introductory discussion of some of the major events related to the history of the disease.
Table 1
Items | Specification |
---|---|
Date of search | October 17th, 2023 |
Databases and other sources searched | PubMed, ClinicalTrials.gov, personal files of authors |
Search terms used | “Thrombotic thrombocytopenic purpura”, “Moschcowitz disease”, and “thrombotic microangiopathy” |
Timeframe | 1924–2023 |
Inclusion and exclusion criteria | Inclusion: peer-reviewed articles |
Exclusion: materials not necessary to convey a brief overview of the history of TTP | |
Selection process | All authors conducted the selection with independent searches |
TTP, thrombotic thrombocytopenic purpura.
Results
Initial discovery and early reports
For many years after Dr. Moschcowitz first published the case report of the unfortunate 16-year-old girl who died of TTP, there was no definitive treatment for the disease, though some patients did respond to infusions of whole blood and plasma exchange; the latter was first attempted in 1956 by Wile and Sturgeon, but did not become standard of care until many decades later (6). In 1959, Dr. Michael Rubinstein and colleagues at what was known at the time as Cedars of Lebanon Hospital in Los Angeles described the case of an 11-year-old girl (7). The case was almost identical to previously published reports of patients with Moschcowitz disease, which was by this point referred to as either TTP or chronic thrombocytopenic purpura. This child presented with 1 week of fever, nausea, vomiting, hematuria, and progressive stupor and confusion. She was pale with petechiae on physical exam, and also had laboratory evidence of hemolysis and thrombocytopenia. Her cognitive status progressively declined. With limited to no options as to how to proceed, the physicians caring for the patient decided to try a whole blood exchange transfusion. Remarkably, the patient’s symptoms reversed, and she was able to be discharged from the hospital. Clearly, there was something in the blood that was central to the pathophysiology of this disease. The thought at the time was that it was likely that either a plasma-related factor was killing these patients or some important yet heretofore unidentified blood component was missing, since giving exogenous whole blood seemed to help reverse the process (Figure 1).
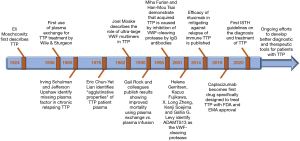
A plasma factor related to disease
A year later in 1960, Schulman et al. explained the reasoning behind this hypothesis (8). They described the case of an 8-year-old German boy who had immigrated to the United States in 1954. The boy had chronic thrombocytopenia, and soon after he was under the care of Dr. Schulman and colleagues in Chicago, he underwent a splenectomy as part of a treatment plan for what was presumed to be idiopathic thrombocytopenic purpura, now known as immune thrombocytopenia (ITP). He did have episodes of severe thrombocytopenia in the years he was followed by the group, calling into question the diagnosis of ITP. When he came in with exacerbations, he was repeatedly treated with whole blood transfusions. These interventions would not only help treat his symptoms, but also led to normal or even high platelet counts. However, the effects of the transfusions on platelet counts only lasted for 2–3 weeks, with the platelet count returning to a baseline of approximately 50,000–70,000 platelets per square millimeter. One of Dr. Schulman’s colleagues, Mila Pierce, speculated that this was because the plasma from the transfusions took about that long to be cleared from the patient’s circulation. Subsequently, they began treating the patient with fresh frozen plasma alone every 3 weeks, with normalization of his platelet counts between transfusions. Analysis of the patient’s family members revealed a genetic pattern for the disease. Dr. Schulman’s paper is a seminal moment in the history of TTP and those who are curious would be well rewarded to spend time reading his remarkable paper.
In 1978, with plasma treatments for patients not yet the standard of care everywhere, Dr. Upshaw, a hematologist at Baptist Memorial Hospital in Memphis, described his experience over 11 years taking care of a patient with the disease, by now known fairly uniformly as TTP (9). Most of the times that she came in with exacerbations, she had both hemolytic anemia and thrombocytopenia. At first, she was treated for exacerbations with whole blood, with prompt resolution of her symptoms and cytopenias; however, he eventually transitioned to using plasma transfusions to treat the patient, with great clinical responses. At this point, it was fairly clear that these patients had some sort of inherited plasma factor deficiency that caused episodes of microangiopathic hemolytic anemia. To this day, patients who have hereditary, or congenital, TTP, are characterized as having Upshaw-Schulman syndrome in honor of the insightful physicians who have helped us to identify these patients and treat them appropriately (10) (Figure 1).
Congenital versus acquired TTP
Unfortunately, even by the mid-1970s, over 50 years after the disease was first described, the majority of patients who presented with signs and symptoms consistent with TTP did not do well (11). Treatment with plasma infusions tended to be most helpful for pediatric patients and those with a hereditary disease pattern, but most patients with TTP were adults with no known hereditary associations. The latter group appeared to have a different pathophysiology of the disease, since plasma transfusions were only partially successful, and improvements in thrombocytopenia for many of these patients lasted for only days, at most (5). The majority of these unfortunate patients died within a few days of diagnosis. To help improve the treatment paradigm for this, Dr. Bukowski and colleagues at the Cleveland Clinic decided to try a different approach (12). They speculated that there may be more than one etiology of TTP, and that at least for some patients, there was a “soluble toxic material” involved in the pathophysiology of the disease. In 1976, Dr. Bukowski reported his experience treating TTP over the past 16 years, with whole blood exchange transfusions in 15 such patients and lasting remissions occurring in nine of them (13). Dr. Rock and other physicians in the Canadian Apheresis Study Group published a landmark trial in 1991 canonizing therapeutic plasma exchange as the gold standard for treatment for TTP, which it remains to be for the acquired form of the disease at the time of this publication (14). They reported the superiority of plasma exchange compared to plasma infusion in terms of mortality. Very few medical interventions in the history of humanity have been shown to be as impactful as therapeutic plasma exchange for the treatment of TTP.
On the other hand, the casual reader may be asking, how do you know whom to treat? And once you decide to treat a patient, to whom do you give simple plasma transfusions and who gets plasma exchange? There are obvious logistical and clinical complications that can arise from either treatment modality. Transfusing any human blood product, including fresh frozen plasma, can lead to immune-mediated reactions, up to and including anaphylaxis and death (15). Plasma exchange for TTP requires the placement of an invasive central catheter in patients with severe thrombocytopenia (16). What if these patients do not need the procedure? Are we not potentially causing unnecessary harm for these patients who may have a completely different reason for their microangiopathic hemolytic anemia and thrombocytopenia with completely different treatments required? The race to answer these questions was on. A deeper understanding of the pathophysiology of the disease was needed.
Insights into the disease mechanism
Towards exploring the pathophysiologic mechanisms that cause TTP, Dr. Lian at the University of Miami reported in 1979 that plasma from patients has “agglutinative properties” (17). Something about the plasma of these patients was causing platelets to aggregate in in vitro studies. It was in 1982 that Dr. Moake at Boston University was able to tell us why platelets were aggregating in these test tubes (18). He noted that the plasma of patients with chronic relapsing TTP in remission had “ultra-large von Willebrand factor (VWF) multimers” and proposed that patients with the disease had an absent “VWF depolymerase”. At long last, the biggest clue as to what was happening to these patients was becoming much clearer. VWF is a large multimeric glycoprotein. We now know, in large part due to the work of Dr. Moake and others, it is a sticky protein that binds to platelets via its A1 domain and helps in the process of primary hemostasis (19). TTP now appeared to be connected to a problem with regulating the size of these multimers of VWF.
As techniques in biochemistry and biology rapidly improved in the 1980s and 1990s, so too would the tools used to help derive further insights into the pathophysiology of many diseases, including TTP. In 1996, the existence of a VWF-cleaving protease (VWF-cp) was identified by Dr. Furlan and colleagues in Bern, as was the cleavage site on VWF in its A2 domain between Tyr1605 and Met1606 (20). These residues corresponded with the VWF peptide bond cleavage site earlier reported in normal plasma and plasma from patients with type IIA von Willebrand disease, a bleeding disorder in which VWF is excessively susceptible to enzymatic degradation (21). In that same year of 1996, Dr. Tsai reported that the effect of VWF-cp was found to be dependent upon shear stress, indicating that when low shear stress is present in the vasculature, VWF remains unaffected by the enzyme (22). Though this finding suggests a role of the conformational environment of VWF on the efficiency of the VWF-cp, both groups confirmed the hypothesis by showing that cleavage of VWF was enhanced in the presence of urea or guanidinium chloride (20,22).
The basic building blocks were in place by that time to explore what was happening in the plasma of patients with TTP. In 1997, Furlan and colleagues reported the interesting findings associated with four patients with chronic relapsing TTP, including two brothers (23). All four patients had ultra-large VWF multimers and constitutional deficiency of protease activity. Mixing the plasma from the patients with normal plasma at a 1:1 ratio showed no inhibition of VWF-cp in normal plasma. These findings were reminiscent of findings in the patient cared for by Dr. Upshaw, and implied that such patients had a hereditary deficiency of VWF-cp.
In contrast, and by extrapolation, simple infusion of a deficient factor would be sufficient if the main mechanism of disease was VWF-cp deficiency. As the reader is already aware, outcomes were significantly better with plasma exchange than with simple plasma infusion. Insights into the pathophysiology of the disease became clearer in 1998. For context, here we will describe a case reported by the team in Bern of a patient with acute TTP who was closely followed over more than 1 year (24). Severe VWF-cp deficiency was diagnosed at the patient’s first bout of TTP. Prolonged plasma exchange, fresh frozen plasma replacement, and steroids were used to treat the patient. The patient was able to enter remission and normalization of VWF-cp. Crucially, identification of an immunoglobulin G (IgG) inhibitor of the VWF-cp was reported in the initial plasma sample. Several months later the patient had another bout of acute TTP with severe VWF-cp deficiency and reappearance of an inhibitor. Once again, treatment led to remission. The patient had a second relapse 330 days after the initial episode, again with severe VWF-cp deficiency. Due to the frequent relapses, splenectomy was performed on day 365, leading to lasting remission and lasting normalization of VWF-cp, as well as lasting disappearance of IgG inhibitor, the first time a VWF-cp inhibitor was described in the literature.
Accumulating evidence was mounting that VWF-cp deficiency was central to the pathophysiology of TTP, and that in the acquired form, IgG inhibitors of VWF-cp were present. Here we acknowledge the insights gained by two groups that looked at larger cohorts of TTP patients to explore this solidifying hypothesis and reported their findings in 1998. Furlan and colleagues obtained plasma samples from 53 patients diagnosed with either TTP or hemolytic uremic syndrome (HUS) and found that none of the HUS patients, but all of the TTP patients, had deficiency of VWF-cp (25). Tsai and Lian, in the same year and published in the same journal on the same day, tested for VWF-cp activity and IgG inhibitor in 39 plasma samples from 37 acquired TTP patients at different points of time during their clinical course (26). Both groups found that VWF-cp was deficient during acute TTP episodes, but not when the patients were in remission nor in the plasma of normal subjects. An inhibitor was detected in the majority of the TTP patients during acute episodes and was identified as IgG in all positive tests by both groups. Crucially, the inhibitor was not detectable when the patients with acquired TTP were in remission from the disease.
At this point, important questions were answered. Some patients with TTP are born with congenital deficiency of VWF-cp. Most, however, had acquired TTP with IgG inhibitors. Therefore, they had an acquired VWF-cp deficiency. These discoveries explained why plasma exchange and plasma replacement are better in acquired TTP, whereas plasma infusion alone is an appropriate treatment for congenital TTP (27).
Discovery of ADAMTS13
One major piece of the puzzle remained. What was the VWF-cp? In 2001, the answer came. The VWF-cp isolated from plasma in 1996 was purified to homogeneity by several groups: Gerritsen et al. in Bern, Fujikawa et al. in Seattle, Soejima et al. in Kumamoto, and Zheng et al. in St. Louis (20,22,28-32). In parallel the group of Dr. Ginsburg reported a genome-wide linkage analysis in four pedigrees with hereditary TTP (33). Amino-acid analysis of the purified protease as well as the genetic analysis found the same gene/protein, i.e., ADAMTS13 on chromosome 9q34 (28-30,32,33). Plaimauer et al. cloned, expressed and functionally characterized active ADAMTS13 in mammalian cells in 2002 (31). Work by Gerhard Antoine and colleagues published in 2003 showed that recombinant ADAMTS13 could be added to the plasma of patients with congenital TTP to rescue VWF-cp activity (34). We now know, from validated clinical assays, that ADAMTS13 deficiency is necessary, but not sufficient, for TTP, and that recovery of ADAMTS13 activity corresponds to resolution of the disease (27,35) (Figure 1).
Modern treatments for TTP
Though this is a large topic which requires its own dedicated focus to fully appreciate, we will briefly summarize the remarkable newer options for TTP treatment that have been discovered and developed in recent years. Insights into the pathophysiology of immune-mediated TTP (iTTP) have led to the standard use of immunosuppressive treatments for these patients, including the monoclonal B-cell inhibitor rituximab (27,36-38). In recent years, caplacizumab, a nanobody that targets the A1 domain of VWF to prevent adhesion of platelets and their clumping in the microvasculature, has been approved by the Food and Drug Administration (FDA) and European Medicines Agency (EMA) for treatment of TTP, the first and only TTP-specific treatment approved to date (39-42). Rituximab significantly reduces the rate of relapse for immune TTP patients, while recent literature suggests a mortality benefit for patients who receive caplacizumab (43-45). There are ongoing clinical trials using recombinant ADAMTS13 both for the treatment of congenital as well as immune TTP (NCT02216084 and NCT03922308) (46). The landscape of treatment for TTP is evolving continuously thanks to the knowledge base built over the last century by investigators in the field.
Conclusions
The journey from a 16-year-old girl who died in the 1920s to the discovery of ADAMTS13 and the consequences of its severe hereditary or acquired deficiency has led us to remarkable realizations about blood and how it works. Having reviewed the broad strokes of the history of TTP here, we have only scratched the surface of what we now know about both ADAMTS13 and TTP. Since the discovery of ADAMTS13, it is now much easier to identify and diagnose patients with TTP. Nonetheless, most centers still require send-out testing for ADAMTS13 which can take up to a week to result (35). In the meantime, many patients with high suspicion of having TTP will undergo plasma exchange with all its concomitant potential complications, only to find out that they do not have TTP. However, with increasingly available rapid testing of ADAMTS13 activity, overtreating patients with thrombotic microangiopathy who do not have TTP will decrease over time.
Perspective
The future of TTP diagnosis and treatment was built on its past, and we all owe a debt of gratitude to the open minds that have led us to this point and beyond. With future discoveries leading to further refinement of our understanding of the function of ADAMTS13; the mechanism of disease in both congenital and immune TTP; and the interplay between ADAMTS13, hemostasis, and the immune system, we will improve how we care for TTP patients. We hope to drastically decrease the time to diagnosis at health centers around the world, as well as generate more targeted therapies that are easier to distribute and administer with fewer adverse sociobiological obstacles.
Acknowledgments
Funding: This work was supported by
Footnote
Provenance and Peer Review: This article was commissioned by the editorial office, Annals of Blood for the series “Thrombotic Thrombocytopenic Purpura”. The article has undergone external peer review.
Reporting Checklist: The authors have completed the Narrative Review reporting checklist. Available at https://aob.amegroups.org/article/view/10.21037/aob-23-46/rc
Peer Review File: Available at https://aob.amegroups.org/article/view/10.21037/aob-23-46/prf
Conflicts of Interest: All authors have completed the ICMJE uniform disclosure form (available at https://aob.amegroups.org/article/view/10.21037/aob-23-46/coif). The series “Thrombotic Thrombocytopenic Purpura” was commissioned by the editorial office without any funding or sponsorship. B.L. reports being chairman of data monitoring committees of studies investigating rADAMTS13 for the treatment of congenital and acquired TTP (Takeda), chairman of the data monitoring committee of a study investigating caplacizumab for the treatment of iTTP without plasma exchange (Sanofi), recipient of congress travel support and/or lecture fees from Baxter, Ablynx, Alexion, Siemens, Bayer, Roche and Sanofi over the past 10 years. X.L.Z. was supported by the NIH and Answering TTP Foundation, he has received grants, including R01 HL144552, R01 HL157975-01A1, and R01 HL164016-01A1. X.L.Z. has received consulting fees from Alexion, Apollo, BioMedica, Argenx, GC Biopharma, Kyowa Kirin, Sanofi, Takeda, he also was paid by Takeda. X.L.Z. is a board member of ISTH VWD subcommittee, he has received other service from Technoclone. X.L.Z. served as the unpaid Guest Editor of the series and serves as an unpaid Editorial Board member of Annals of Blood from March 2022 to February 2024. K.H. is supported by the NIH grant HL163471 and a Career Development Award from the American Heart Association. The authors have no other conflicts of interest to declare.
Ethical Statement: The authors are accountable for all aspects of the work in ensuring that questions related to the accuracy or integrity of any part of the work are appropriately investigated and resolved.
Open Access Statement: This is an Open Access article distributed in accordance with the Creative Commons Attribution-NonCommercial-NoDerivs 4.0 International License (CC BY-NC-ND 4.0), which permits the non-commercial replication and distribution of the article with the strict proviso that no changes or edits are made and the original work is properly cited (including links to both the formal publication through the relevant DOI and the license). See: https://creativecommons.org/licenses/by-nc-nd/4.0/.
References
- Moschcowitz E. An acute febrile pleiochromic anemia with hyaline thrombosis of the terminal arterioles and capillaries: an undescribed disease. Arch Intern Med 1925;36:89-93. [Crossref]
- Moschcowitz ELI. Hyaline thrombosis of the terminal arterioles and capillaries: a hitherto undescribed disease. Proc NY Pathol Soc 1924;24:21-4.
- Singer K, Bornstein FP, Wile SA. Thrombotic thrombocytopenic purpura; hemorrhagic diathesis with generalized platelet thromboses. Blood 1947;2:542-54. [Crossref] [PubMed]
- Evans RS, Takahashi K, Duane RT, et al. Primary thrombocytopenic purpura and acquired hemolytic anemia; evidence for a common etiology. AMA Arch Intern Med 1951;87:48-65. [Crossref] [PubMed]
- Amorosi EL, Ultmann JE. Thrombotic thrombocytopenic purpura: report of 16 cases and review of the literature. Medicine 1966;45:139-60. [Crossref]
- Wile SA, Sturgeon P. Thrombotic thrombocytopenic purpura; review of the subject with a report of three cases in children. Pediatrics 1956;17:882-96. [Crossref] [PubMed]
- Rubinstein MA, Kagan BM, Macgillviray MH, et al. Unusual remission in a case of thrombotic thrombocytopenic purpura syndrome following fresh blood exchange transfusions. Ann Intern Med 1959;51:1409-19. [Crossref] [PubMed]
- Schulman I, Pierce M, Lukens A, et al. Studies on thrombopoiesis. I. A factor in normal human plasma required for platelet production; chronic thrombocytopenia due to its deficiency. Blood 1960;16:943-57. [Crossref] [PubMed]
- Upshaw JD Jr. Congenital deficiency of a factor in normal plasma that reverses microangiopathic hemolysis and thrombocytopenia. N Engl J Med 1978;298:1350-2. [Crossref] [PubMed]
- Rennard S, Abe S. Decreased cold-insoluble globulin in congenital thrombocytopenia (Upshaw-Schulman syndrome). N Engl J Med 1979;300:368. [Crossref] [PubMed]
- Umlas J, Kaiser J. Thrombohemolytic thrombocytopenic purpura (TTP). A disease or a syndrome? Am J Med 1970;49:723-8. [Crossref] [PubMed]
- Bukowski RM, King JW, Hewlett JS. Plasmapheresis in the treatment of thrombotic thrombocytopenic purpura. Blood 1977;50:413-7. [Crossref] [PubMed]
- Bukowski RM, Hewlett JS, Harris JW, et al. Exchange transfusions in the treatment of thrombotic thrombocytopenic purpura. Semin Hematol 1976;13:219-32. [PubMed]
- Rock GA, Shumak KH, Buskard NA, et al. Comparison of plasma exchange with plasma infusion in the treatment of thrombotic thrombocytopenic purpura. Canadian Apheresis Study Group. N Engl J Med 1991;325:393-7. [Crossref] [PubMed]
- Irani MS, Sanchez F, Friedman K. Caplacizumab for treatment of thrombotic thrombocytopenic purpura in a patient with anaphylaxis to fresh-frozen plasma. Transfusion 2020;60:1666-8. [Crossref] [PubMed]
- Rizvi MA, Vesely SK, George JN, et al. Complications of plasma exchange in 71 consecutive patients treated for clinically suspected thrombotic thrombocytopenic purpura-hemolytic-uremic syndrome. Transfusion 2000;40:896-901. [Crossref] [PubMed]
- Lian EC, Harkness DR, Byrnes JJ, et al. Presence of a platelet aggregating factor in the plasma of patients with thrombotic thrombocytopenic purpura (TTP) and its inhibition by normal plasma. Blood 1979;53:333-8. [Crossref] [PubMed]
- Moake JL, Rudy CK, Troll JH, et al. Unusually large plasma factor VIII: von Willebrand factor multimers in chronic relapsing thrombotic thrombocytopenic purpura. N Engl J Med 1982;307:1432-5. [Crossref] [PubMed]
- Ginsburg D, Sadler JE. von Willebrand disease: a database of point mutations, insertions, and deletions. For the Consortium on von Willebrand Factor Mutations and Polymorphisms, and the Subcommittee on von Willebrand Factor of the Scientific and Standardization Committee of the International Society on Thrombosis and Haemostasis. Thromb Haemost 1993;69:177-84. [Crossref] [PubMed]
- Furlan M, Robles R, Lämmle B. Partial purification and characterization of a protease from human plasma cleaving von Willebrand factor to fragments produced by in vivo proteolysis. Blood 1996;87:4223-34. [Crossref] [PubMed]
- Dent JA, Berkowitz SD, Ware J, et al. Identification of a cleavage site directing the immunochemical detection of molecular abnormalities in type IIA von Willebrand factor. Proc Natl Acad Sci U S A 1990;87:6306-10. [Crossref] [PubMed]
- Tsai HM. Physiologic cleavage of von Willebrand factor by a plasma protease is dependent on its conformation and requires calcium ion. Blood 1996;87:4235-44. [Crossref] [PubMed]
- Furlan M, Robles R, Solenthaler M, et al. Deficient activity of von Willebrand factor-cleaving protease in chronic relapsing thrombotic thrombocytopenic purpura. Blood 1997;89:3097-103. [Crossref] [PubMed]
- Furlan M, Robles R, Solenthaler M, et al. Acquired deficiency of von Willebrand factor-cleaving protease in a patient with thrombotic thrombocytopenic purpura. Blood 1998;91:2839-46. [Crossref] [PubMed]
- Furlan M, Robles R, Galbusera M, et al. von Willebrand factor-cleaving protease in thrombotic thrombocytopenic purpura and the hemolytic-uremic syndrome. N Engl J Med 1998;339:1578-84. [Crossref] [PubMed]
- Tsai HM, Lian EC. Antibodies to von Willebrand factor-cleaving protease in acute thrombotic thrombocytopenic purpura. N Engl J Med 1998;339:1585-94. [Crossref] [PubMed]
- Zheng XL, Vesely SK, Cataland SR, et al. ISTH guidelines for treatment of thrombotic thrombocytopenic purpura. J Thromb Haemost 2020;18:2496-502. [Crossref] [PubMed]
- Fujikawa K, Suzuki H, McMullen B, et al. Purification of human von Willebrand factor-cleaving protease and its identification as a new member of the metalloproteinase family. Blood 2001;98:1662-6. [Crossref] [PubMed]
- Zheng X, Chung D, Takayama TK, et al. Structure of von Willebrand factor-cleaving protease (ADAMTS13), a metalloprotease involved in thrombotic thrombocytopenic purpura. J Biol Chem 2001;276:41059-63. [Crossref] [PubMed]
- Soejima K, Mimura N, Hirashima M, et al. A novel human metalloprotease synthesized in the liver and secreted into the blood: possibly, the von Willebrand factor-cleaving protease? J Biochem 2001;130:475-80. [Crossref] [PubMed]
- Plaimauer B, Zimmermann K, Völkel D, et al. Cloning, expression, and functional characterization of the von Willebrand factor-cleaving protease (ADAMTS13). Blood 2002;100:3626-32. [Crossref] [PubMed]
- Gerritsen HE, Robles R, Lämmle B, et al. Partial amino acid sequence of purified von Willebrand factor-cleaving protease. Blood 2001;98:1654-61. [Crossref] [PubMed]
- Levy GG, Nichols WC, Lian EC, et al. Mutations in a member of the ADAMTS gene family cause thrombotic thrombocytopenic purpura. Nature 2001;413:488-94. [Crossref] [PubMed]
- Antoine G, Zimmermann K, Plaimauer B, et al. ADAMTS13 gene defects in two brothers with constitutional thrombotic thrombocytopenic purpura and normalization of von Willebrand factor-cleaving protease activity by recombinant human ADAMTS13. Br J Haematol 2003;120:821-4. [Crossref] [PubMed]
- Zheng XL, Vesely SK, Cataland SR, et al. ISTH guidelines for the diagnosis of thrombotic thrombocytopenic purpura. J Thromb Haemost 2020;18:2486-95. [Crossref] [PubMed]
- Uhl L, Kiss JE, Malynn E, et al. Rituximab for thrombotic thrombocytopenic purpura: lessons from the STAR trial. Transfusion 2017;57:2532-8. [Crossref] [PubMed]
- Fakhouri F, Vernant JP, Veyradier A, et al. Efficiency of curative and prophylactic treatment with rituximab in ADAMTS13-deficient thrombotic thrombocytopenic purpura: a study of 11 cases. Blood 2005;106:1932-7. [Crossref] [PubMed]
- Jestin M, Benhamou Y, Schelpe AS, et al. Preemptive rituximab prevents long-term relapses in immune-mediated thrombotic thrombocytopenic purpura. Blood 2018;132:2143-53. [Crossref] [PubMed]
- Scully M, Cataland SR, Peyvandi F, et al. Caplacizumab Treatment for Acquired Thrombotic Thrombocytopenic Purpura. N Engl J Med 2019;380:335-46. [Crossref] [PubMed]
- Peyvandi F, Scully M, Kremer Hovinga JA, et al. Caplacizumab reduces the frequency of major thromboembolic events, exacerbations and death in patients with acquired thrombotic thrombocytopenic purpura. J Thromb Haemost 2017;15:1448-52. [Crossref] [PubMed]
- Peyvandi F, Scully M, Kremer Hovinga JA, et al. Caplacizumab for Acquired Thrombotic Thrombocytopenic Purpura. N Engl J Med 2016;374:511-22. [Crossref] [PubMed]
- Holz JB. The TITAN trial--assessing the efficacy and safety of an anti-von Willebrand factor Nanobody in patients with acquired thrombotic thrombocytopenic purpura. Transfus Apher Sci 2012;46:343-6. [Crossref] [PubMed]
- Kubo M, Sakai K, Yoshii Y, et al. Rituximab prolongs the time to relapse in patients with immune thrombotic thrombocytopenic purpura: analysis of off-label use in Japan. Int J Hematol 2020;112:764-72. [Crossref] [PubMed]
- Goyal J, Adamski J, Lima JL, et al. Relapses of thrombotic thrombocytopenic purpura after treatment with rituximab. J Clin Apher 2013;28:390-4. [Crossref] [PubMed]
- Peyvandi F, Cataland S, Scully M, et al. Caplacizumab prevents refractoriness and mortality in acquired thrombotic thrombocytopenic purpura: integrated analysis. Blood Adv 2021;5:2137-41. [Crossref] [PubMed]
- Scully M, Knöbl P, Kentouche K, et al. Recombinant ADAMTS-13: first-in-human pharmacokinetics and safety in congenital thrombotic thrombocytopenic purpura. Blood 2017;130:2055-63. [Crossref] [PubMed]
Cite this article as: Halkidis K, Lämmle B, Zheng XL. The history of thrombotic thrombocytopenic purpura research: a narrative review. Ann Blood 2024;9:16.