Challenges with assigning RH alleles and accurately predicting phenotypes using commercially-available genotyping kits: a narrative review
Introduction
Background
Utility of molecular methods for predicting phenotype
Molecular methods have been used to catalogue (1) and explore blood group antigen genes and predict phenotypes for more than three decades (2-4) including a focus on the RH blood group system (5). There are multiple patient use cases for molecular immunohematology including after recent transfusion, when serologic reagents are not available, to resolve antigen typing discrepancies, to aid in complex antibody identifications, to assess the risk of alloimmunization including hemolytic disease of the fetus and newborn (HDFN) and fetal and neonatal alloimmune thrombocytopenia (FNAIT) as well as to determine zygosity status. Use cases for molecular immunohematology in blood donors include to efficiently predict multiple antigen phenotypes in multiple individuals simultaneously as well as to resolve antigen typing discrepancies and to assign RH alleles for purposes of RH allele matching (6-10). These use cases have been reviewed elsewhere (11).
The RH Blood Group System (ISBT 004)
The RH blood group system is encoded by two highly homologous genes (RHD and RHCE), both with 10 exons. The RHD gene encodes one common antigen, RhD or RH1, while the RHCE gene encodes two sets of antithetical common antigens, big C (RH2) and little c (RH4) and big E (RH3) and little e (RH5). Rare phenotypes include Rhnull with loss of all RH antigens (12), and D—, D.. (13) and those with loss of high prevalence antigens including hrB (RH31) and hrS (RH19) (14). Importantly, there are multiple genetic backgrounds that can result in loss of these antigens (15).
Rationale and knowledge gap
The purpose of this narrative review is to provide the reader with an understanding of how a molecular test used to predict RH blood group system phenotypes can yield an incorrect or equivocal result. The understanding of test limitations can be helpful in interpreting the results and determining if additional testing is warranted. This information is useful to molecular and serologic immunohematology reference laboratories as well as blood centers and hospital blood banks.
Objective
The objective of this review is to address the following questions
- What is the impact of resolution of genotyping methods on genotype-predicted phenotype?
- What red cell genotyping panels are commercially-available?
- What are the limitations of commercially-available red cell genotyping panels, including:
- Failure to test for variants associated with most null alleles;
- Failure to test for uncommon but clinically significant variants;
- Failure to test for common variants with impact on predicted phenotype.
- What is the impact of inferences due to linkage disequilibrium and phase assumptions?
- What is the impact of equivocal allele assignments on antigen phenotypes?
I present this article in accordance with the Narrative Review reporting checklist (available at https://aob.amegroups.com/article/view/10.21037/aob-23-18/rc).
Methods
Peer-reviewed literature with key words RH, genotyping, limitations, antigen and blood published in English in PubMed from 1990 to April 2023 was reviewed (Table 1). Commercial genotyping kit package inserts were reviewed including Immucor PreciseType HEA Molecular BeadChip, RHD and RHCE BeadChip, Grifols IDCoreXT. ISBT Working Party for Red Cell Immunogenetics and Blood Group Terminology allele tables for RHD, RHCE and FY were reviewed (1). Insights were derived from more than a decade managing a national reference laboratory of molecular immunohematology.
Table 1
Items | Specification |
---|---|
Date of search | April 29, 2023 |
Database searched | PubMed |
Search terms used | RH, genotyping, limitations, antigen, blood |
Timeframe | 1990 to April 2023 |
Inclusion criteria | Peer-reviewed literature published in English |
Selection process | Selection conducted by the author |
Any additional considerations | Commercial genotyping kit package inserts were reviewed including Immucor PreciseType HEA Molecular BeadChip, RHD and RHCE BeadChip, Grifols IDCoreXT |
ISBT Working Party for Red Cell Immunogenetics and Blood Group Terminology allele tables for RHD, RHCE and FY were reviewed | |
RHD blood group alleles v6.3 31-MAR-2023 (accessed on 09/04/2023) | |
RHD blood group alleles v6.4 31-JUL-2023 (accessed on April 29, 2023) | |
RHCE blood group alleles v6.2 31-MAR-2022 (accessed on April 29, 2023) | |
FY blood group alleles v6.1 30-NOV-2021 (accessed on April 29, 2023) | |
Insights were derived from more than a decade managing a national reference laboratory of molecular immunohematology |
Table 1 summarizes the literature search strategy used to gather information to include in this review.
Findings
Genotyping test resolution
Blood group antigens are determined by genetic variation, including copy number variants, insertion/deletion polymorphisms and single nucleotide variants (SNV). Currently, commercial genotyping panels that include prediction of RH antigens are based on SNV typing. Molecular testing methods can be classified as low-, medium- or high resolution. High resolution methods include those that interrogate all critical gene regions such as coding regions and splice sites. Examples of high-resolution test methods are Sanger sequencing (16) and next-generation sequencing (17). Though there are several reports of targeted NextGen panels for blood group antigen expression, currently, there are no commercial high-resolution kits for predicting red cell antigens including RH antigens. Low-resolution genotyping is characterized by using one or a limited set of markers to predict antigen expression. An example would be the PreciseType HEA Molecular BeadChip (Immucor, Norcross, GA, USA), that predicts RH antigen expression but does not include sufficient markers to detect variant antigens. Medium-resolution genotyping is characterized by inclusion of markers sufficient to detect common variants. Examples include the RHCE and RHD BeadChips (Immucor) that includes 25 and 35 genetic markers, respectively. A limitation of both low-and medium-resolution panels is the failure to detect variants that can impact antigen expression. These can be variants that result in weak or partial antigen expression, gain of low-prevalence antigen expression or loss of high prevalence antigen expression.
Available genotyping products that include RH antigens
All currently commercially available genotyping panels that predict RH blood group system antigens are low or medium resolution. Table 2 lists the commercially-available genotyping panels that include RH antigens. Only two are approved for use by the US Food and Drug Administration (FDA), several are Conformité Européénne (CE)-marked and several are sold as “research use only” (RUO). These include bead-based fluorescence assays from Immucor and Grifols (Barcelona, Spain), as well as sequence-specific primer (SSP)-polymerase chain reaction (PCR)-based assays from inno-Train diagnostic GMBH (Kronberg, Germany) and BAG Diagnostics (Lich, Germany), as well as Matrix-assisted laser desorption ionization-time-of-flight (MALDI-TOF) mass spectrometry from Agena Bioscience (San Diego, CA, USA).
Table 2
Test name | Manufacturer | Methodology | Approval |
---|---|---|---|
PreciseType HEA Molecular BeadChip | Immucor | Elongation-mediated Multiplexed Analysis of Polymorphisms (eMAP®) | FDA, CE certified |
IDCoreXT | Grifols | Qualitative, PCR and hybridization-based genotyping test | FDA, CE certified |
RHD BeadChip | Immucor | Elongation-mediated Multiplexed Analysis of Polymorphisms (eMAP®) | CE certified |
RHCE BeadChip | Immucor | Elongation-mediated Multiplexed Analysis of Polymorphisms (eMAP®) | CE certified |
ID RHD XT | Grifols | Qualitative, PCR and hybridization-based genotyping test | RUO |
HemoID DQS | Agena BioScience | MALDI-TOF mass spectrometry | RUO |
RBC-FluoGene | Inno-train diagnostic GMBH | TaqMan-based SSP-assay | CE certified |
RBC-ReadyGene | Inno-train diagnostic GMBH | PCR-SSP-assay | CE certified |
BAGene | BAG diagnostics | PCR-SSP | CE certified |
FDA, Food and Drug Administration; CE, Conformité Européénne; PCR, polymerase chain reaction; RUO, research use only; SSP, sequence-specific primer; MALDI-TOF, matrix-assisted laser desorption ionization-time-of-flight.
Test limitations
The next sections will present examples of limitations of low and medium-resolution genotyping panels.
What’s missing on panels: null alleles
Most low-resolution commercial panels and many medium resolution commercial panels do not include analytes that detect null alleles. This can lead to false positive predicted phenotypes. An example is found in the RH blood group system, specifically in the RHCE gene. A sample may be tested for the common antigens C, c, E and e using a low-resolution commercial RBC genotyping panel and predicted to express all of them. Even a medium-resolution genotyping panel focused on RHCE markers might be performed and would also predict expression of C, c, E and e. If the RBCs test C+ e+ c− E−, a null allele should be suspected since each RHCE allele would be expected to express one of each antithetical antigen pair (C/c, E/e). High-resolution testing can be used to rule this scenario in or out. A false positive prediction for c and E antigen expression would be expected in a sample carrying one RHCE*Ce allele and the other RHCE*cEN.02 allele that includes a single nucleotide deletion associated with premature termination (18).
What’s missing on panels: uncommon variants
Antigen typing discrepancies can identify samples that may carry variant antigens that are expressed weakly and/or lack epitopes that result in differential reactivity with serologic reagents. RhD is the classic example (19). There are several medium-resolution commercial RHD genotyping panels. These panels are generally assembled to cover common variants and often are selected based on findings in Caucasian and in some cases populations of African descent. Use of these panels may result in failure to identify genetic variants that are associated with weakened antigen expression or loss of epitopes. This is a serious limitation when this information is used to determine candidacy for Rh immune prophylaxis in women of child-bearing age (20). An example of this is a female with trauma found to have differential reactivity with two anti-D reagents. The sample was tested using a medium resolution panel that interrogates 35 genetic variants. The patient was found to have no variants and predicted to type D+ and based on that result, would not be expected to be at risk of allo-anti-D formation. However, high-resolution Sanger sequencing was performed of all coding exons and the patient was found to carry RHD c.1195A associated with RHD*01W.45. This allele has been reported to be associated with allo-anti-D (21). This case highlights the need for caution when a medium-resolution testing platform does not resolve a serologic discrepancy. Such cases should be referred to a reference laboratory where high resolution testing can be performed.
What’s missing on panels: common variants
There are several examples of common variants not included on commercial genotyping panels having an impact on the interpretation of the results.
One example is in RHCE, where the c.48G>C SNV (rs586178) is very common. It has a global minor allele frequency of 0.40 and a minor allele 0.20 in African Americans. RHCE c.48C is found on RHCE alleles that express the big C antigen as well as many variant RHCE*ce alleles (see Table 3). The presence of RHCE c.48C changes the phenotype in some instances, as shown in Table 4. The impact of c.48C alone is associated with a weak e phenotype as per ISBT (1) but has been found in individuals with allo-anti-e (22).
Table 3
RHCE allele name/RHCE allele alias | Nucleotides | Phenotype† |
---|---|---|
RHCE*01.01/RHCE*ce48C | c.48G>C | RH:5 (e+ weak) |
RHCE*01.02/RHCE*ceTI | c.48G>C c.1025C>T |
RH:4 (c+ weak, partial) |
RH:5 (e+ weak, partial) | ||
RHCE*01.04/RHCE*ceAR | c.48G>C | RH:4 (c+ partial) |
c.712A>G | RH:5 (e+ weak, partial) | |
c.733C>G | RH:10,–20 (V+ weak, VS–) | |
c.787A>G | RH:–18,–19 (Hr–, hrS–) | |
c.800T>A | ||
c.916A>G | ||
RHCE*01.05/RHCE*ceEK | c.48G>C | RH:4 (c+ partial) |
c.712A>G | RH:5 (e+ weak, partial) | |
c.787A>G | RH:–18,–19 (Hr–, hrS–) | |
c.800T>A | ||
RHCE*01.07/RHCE*ceMO | c.48G>C | RH:4 (c+ partial) |
c.667G>T | RH:5 (e+ weak, partial) | |
RH:–19 (hrS–) | ||
RH:–31 (hrB–) | ||
RH:–61 (CEVF–) | ||
RHCE*01.08/RHCE*ceBI | c.48G>C | RH:5 (e+ partial, weak to neg) |
c.712A>G | RH:–18,–19 (Hr–, hrS–) | |
c.818C>T | RH:49 (STEM+) | |
c.1132C>G | ||
RHCE*01.09/RHCE*ceSM | c.48G>C | RH:5 (e+ positive to negative) |
c.712A>G | RH:–18 (Hr–), inferred | |
c.818C>T | RH:–19 (hrS–) | |
Rh:49 (STEM+ weak) | ||
RHCE*01.10/RHCE*ceSL | c.48G>C | RH:5 (e+ weak) |
c.365C>T | Some monoclonal anti-D crossreact | |
RHCE*01.12/RHCE*ceRA | c.48G>C | RH:5 (e+ weak) |
c.538G>C | ||
RHCE*01.16/RHCE*ce.16 | c.48G>C | RH:5 (e+ weak) |
c.1170C>T | ||
c.1193T>A | ||
RHCE*01.20.02/RHCE*ceVS.02 | c.48G>C | RH:4 (c+ partial) |
c.733C>G | RH:5 (e+ partial) | |
RH:10,20 (V+VS+) | ||
RH:–31 (hrB–) | ||
RHCE*01.20.03/RHCE*ceVS.03 | c.48G>C | RH:4 (c+ partial) |
c.733C>G | RH:5 (e+ partial) | |
c.1006G>T | RH:–10,20 (V–VS+) | |
RH:–31 (hrB–) | ||
RHCE*01.20.04/RHCE*ceTI Type 2 | c.48G>C | RH:5 (e+ partial) |
c.733C>G | RH:10,20 (V+VS+) | |
c.1025C>T | Probable RH:–31 (hrB–) | |
RHCE*01.20.06/RHCE*ceCF | c.48G>C | RH:4 (c+ partial) |
c.697C>G | RH:5 (e+ partial, positive to negative) | |
c.733C>G | RH:20 (VS+) | |
RH:–19,–31 (hrS–, hrB–) | ||
RH:43 (Crawford+) | ||
RH:–58 (CELO–) | ||
Some monoclonal anti-D crossreact | ||
RHCE*01.20.08/RHCE*ceVS.08 | c.48G>C | RH:5 (e+ weak) |
c.733C>G | RH:10,20 (V+VS+) | |
c.748G>A | Probable RH:–31 (hrB–) | |
RHCE*01.20.09/RHCE*ceVS.09 | c.48G>C | RH:5 (e+ weak) |
c.733C>G | RH:10,20 (V+VS+) | |
c.941T>C | RH:31 (hrB+ weak) | |
RHCE*01.20.10/RHCE*ceVS.10 | c.48G>C | Probable RH:4 (c+ partial) |
c.712A>G | Probable RH:5 (e+ partial) | |
c.733C>G | ||
RHCE*01.20.11/RHCE*ceVS.11 | c.48G>C | Not reported |
exons 2-3 D | ||
c.186G>T | ||
c.410C>T | ||
c.455A>C | ||
c.733C>G | ||
c.1006G>T | ||
RHCE*01.20.12/RHCE*ceVS.12 | c.48G>C | Some monoclonal anti-D crossreact |
c.462G>T | ||
c.733C>G | ||
c.1006G>T | ||
RHCE*01.20.13/RHCE*ceVS.13 | c.48G>C | RH:5 (e+) |
c.106G>A | RH:9 (CX+) | |
c.733C>G | RH:20 (VS+) | |
RHCE*01.21.02/RHCE*ce.21.02 | c.48G>C | RH:5 (e+ weak) |
c.187G>C | RH:48 (JAL+) | |
c.341G>A |
†, “weak” and “partial” phenotypes are not mutually exclusive, and for many alleles the associated phenotype(s) have not been extensively investigated or samples have not been informative. All partial antigens may not be indicated.
Table 4
RHCE allele name | RHCE allele alias | Nucleotides | Phenotype |
---|---|---|---|
RHCE*01 | RHCE*ce | N/A | RH:4 or c |
RH:5 or e | |||
RH:6 or f (ce) | |||
RHCE*01.01 | RHCE*ce48C | c.48G>C | RH:5 (e+ weak) |
RHCE*01.20.01 | RHCE*ceVS.01 | c.733C>G | RH:4 (c+ partial) |
RH:5 (e+ partial) | |||
RH:10,20 (V+VS+) | |||
RH:31 (hrB+ very weak to neg) | |||
RHCE*01.20.02 .01 | RHCE*ceVS.02 .01 | c.48G>C | RH:4 (c+ partial) |
c.733C>G | RH:5 (e+ partial) | ||
RH:10,20 (V+VS+) | |||
RH:–31 (hrB–) | |||
RHCE*01.20.03 | RHCE*ceVS.03 | c.48G>C | RH:4 (c+ partial) |
c.733C>G | RH:5 (e+ partial) | ||
c.1006G>T | RH:–10,20 (V–VS+) | ||
RH:–31 (hrB–) | |||
RHCE*01.20.05 | RHCE*ceVS.05 | c.733C>G | RH:5 (e+ partial) |
c.1006G>T | RH:–10,20 (V–VS+) | ||
RH:–31 (hrB–) | |||
RHCE*01.02.01 | RHCE*ceTI | c.48G>C | RH:4 (c+ weak, partial) |
c.1025C>T | RH:5 (e+ weak, partial) | ||
RHCE*01.03 | RHCE*ce.03 | c.1025C>T | RH:5 (e+ partial) |
N/A, not applicable.
Therefore, when genotyping panels such as IDCore XT (Grifols) fail to test for RHCE c.48C, this may result in incorrect or incomplete predicted phenotypes. This is important in cases of RH allele matching (9,10), when RBC units are selected based on similarity to the patient RH alleles. As described in Keller 2022, donors are ranked based on the similarity of RH alleles between them and a specific patient (23). Tier 1 is the rank given to donors with probable RH alleles that are the same as the probable alleles assigned to the patient. Tier 2 is the rank given to donors homozygous for one of the probable alleles of the patient. Tier 3 is the rank given to donors that carry one or both alleles that are distinct from those carried by the patient but with a similar phenotype. Donors that are ranked Tier 3 often carry RH alleles with additional amino acid substitutions that could have immunogenetic impact. An RBC unit from a donor carrying RHCE*ceEK/RHCE*ceMO would be assigned Tier 3 for a patient with RHCE*ceEK/RHCE*ceEK. Both patient and donor RBCs would be predicted to lack hrS and have a similar phenotype, but the donor red cells express an Rhce molecule with one amino acid (p.223Phe) not expressed by the patient.
The term “probable RH allele” is used to emphasize that the alleles are assigned based on medium-resolution genotyping and therefore carry less than 100% certainty. A case example of how a limitation in the coverage of a genotyping panel impacts selection of donors based on RH allele information involves a patient for which IDCore XT (Grifols) assigns a result of RHCE*ce[733G]/RHCE*ce[733G]. However, when the sample is genotyped using another platform that includes the RHCE c.48G>C SNV, the patient was found to be RHCE*ce48C,733G/RHCE*ce733G. Though the phenotype is the same in this case, this information is useful when performing RH allele matching, and resulted in additional donors being classified as Tier 1 or Tier 2 for this patient.
What’s missing on panels: uncommon but consequential variants
Medium-resolution RH genotyping panels do not rule in or out uncommon variants and this limitation should be noted in testing reports and understood by testing laboratory leadership and hospital clients. A case example is an African American patient whose red cells typed e+ E+. The immunohematology reference lab identified a possible anti-hrB. The commercial RHCE genotyping panel identified no variants. Based on this result, the patient would be predicted to carry RHCE*cE and RHCE*ce alleles. A PCR-restriction fragment length polymorphism (PCR-RFLP) was performed to interrogate an SNV at RHCE c.254C>G (rs57992529) and found the patient to be heterozygous. With this additional information, the allele assignment is RHCE*cE/RHCE*ce01.06.01 and the predicted phenotype is C− c+ E+ partial e+ RH:–31 (hrB–) (24). According to the ALFA project and listed in NCBI (25) this SNV has a global minor allele frequency (MAF) of 0.075 while the MAF in African Americans is 0.2%.
Another gap is found in the commonly used RHD genotyping panels that do not interrogate sufficient SNVs to differentiate some of the DAR family of alleles from Weak D 4.0. Cases of anti-D in a D+ patient where the antibody shows properties of an allo-antibody and/or the patient is of African descent may also benefit from RHD genotyping. Determination of a partial D phenotype is especially critical in patients who are likely to be multiply transfused, such as those with sickle cell disease.
Inferences due to linkage disequilibrium
The analysis software of a commercial genotyping platform that includes RHCE markers uses linkage disequilibrium between RHCE and RHD to provide interpretation that a specimen may carry the (C)ces haplotype. The (C)ces or r’s haplotype is made up of the RHD*03N.01 (RHD*DIIIa-CE(4-7)-D) linked to the RHCE*01.20.03 allele carrying c.48C, c.733G and c.1006T. This haplotype is important due to the loss of D antigen expression and the expression of an altered C antigen by the RHD*03N.01 allele as well as the loss of RH:31 (hrB) and RH:34 (HrB) antigens. However, the accuracy of the prediction is not 100% since the RHCE*01.20.03 allele is also linked to the RHD*03.01 (RHD*DIIIa) allele that encodes a partial D phenotype. Therefore, this information is best used to identify samples for which additional molecular testing is warranted. In a D+ patient carrying RHCE*01.20.03, RHD genotyping would be recommended to rule out co-inheritance with RHD*03.01, a partial D phenotype and risk of allo-anti-D. In a D− patient carrying RHCE*01.20.03, RHD genotyping would be recommended to rule out co-inheritance with RHD*03N.01 and expression of an altered C antigen that could put the patient at risk of allo-anti-C.
Phase assumptions
Currently, prediction of RBC antigen expression primarily utilizes low resolution SNV detection. When multiple SNVs are detected on the same gene, phase is imputed. Commercial genotyping kits utilize software algorithms to predict phenotypes. These algorithms use allele frequency information to make the most likely prediction. Probably the most classic example of this phenomenon is found in the FY blood group system (26), where most commercial assays include not only the c.125G>A SNV that predicts Fya and Fyb expression, but also the SNV for the GATA box variant found in the promoter region. The FY c.-67t>c variant destroys the binding site for the GATA-1 transcription factor that is essential for expression of the FY antigens in red cells (27). This variant is common in individuals of African descent and is primarily found on the FY*02 allele that encodes Fyb; this allele is FY*02N.01. However, it has been found on the FY*01 allele in other populations including in Papua New Guinea where it has been shown to impact Fya expression (28); that allele is FY*01N.01. In samples found to be compound heterozygous for FY c.-67t/c and c.125G/A, though it would be possible for the GATA mutation to be found on the allele encoding Fya and therefore silence Fya, it is unlikely in most populations (see Table 5). Another example in the FY system of phase assumptions in genotyping panel software involves the other common variant in the FY system, Fyx. While the FY c.265C>T SNV is typically inherited on the FY*B allele, it has been found on the FY*A allele (29). Since phase of SNVs is imputed on most genotyping panels, it is important for laboratories performing RBC genotyping using commercial kits to understand their population as well as what algorithms are used by the software to make allele assignments and predict phenotypes.
Table 5
FY c.67t/c | FY c.125G/A | FY c.265C/T | Allele assignment | Predicted phenotype | Comment |
---|---|---|---|---|---|
t/t | G/A | C/C | FY*01/FY*02 | Fy(a+b+) | – |
c/c | A/A | C/C | FY*02N.01/FY*02N.01 | Fy(a−b−) | – |
t/c | G/A | C/C | FY*01/FY*02N.01 | Fy(a+b−) | Likely allele assignment in most populations |
t/c | G/A | C/C | FY*01N.01/FY*02 | Fy(a−b+) | Unlikely but possible allele assignment |
t/t | G/A | C/T | FY*01/FY*02W.01 | Fy(a+b+w) | – |
t/t | G/A | C/T | FY*01W.01/FY*02 | Fy(a+wb) | Unlikely but possible allele assignment |
Equivocal allele assignments due to limitations of SNV genotyping in the context of gene conversion
Medium-resolution RHD genotyping, especially in individuals of African descent, can result in equivocal allele assignments due to inability to determine if exons in one allele failed to generate an amplicon due to presence of a hybrid allele. There are many RHD alleles where a portion of the gene, including one or several exons, have been replaced with RHCE sequences due to a gene conversion event. Since the genotyping panel uses gene-specific amplification of each exon in which SNVs on the panel are detected (Figure 1), this can result in “allele dropout”. The most common example is the inability to determine if a sample carries the RHD*03.01 (RHD*DIIIa) allele in homozygosity (Figure 2A) or hemizygosity (Figure 2B) or if it is a compound heterozygous for RHD*03.01 and the RHD*03N.01 (RHD*DIIIa-CE(4-7)-D) (Figure 2C). In the case of this allele combination, exons 4–7 are amplified only from the RHD*03.01 allele since exons 4–7 of RHD*03N.01 are derived from RHCE and the primer pairs fail to amplify a product.
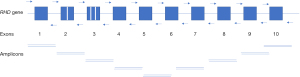
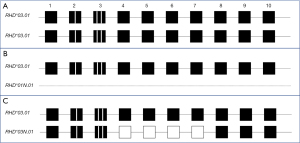
Differentiating the allele combination in Figure 2B,2C cannot be deduced based on SNV typing alone since both RHD*03.01 and RHD*03N.01 carry RHD c.186G>T, c.410C>T, c.455A>C SNVs. The impact on phenotype is significant since RHD*03.01 encodes a partial D phenotype and expression of the low-prevalence antigen DAK while RHD*03N.01 does not encode the D antigen but instead encodes an altered C antigen. Individuals carrying this allele type C+ but are at risk of making allo-anti-C. While the individuals with RHD allele combinations shown in Figure 2A,2B would express a partial D phenotype, the individual with RHD allele combinations shown in Figure 2C would express a partial D phenotype as well as an altered C antigen. If serologic typing for C antigen is available or can be performed, the serologic information can be paired with the genotyping results to assign the alleles and predicted phenotype more accurately.
Equivocal allele assignments due to compound heterozygosity
Medium-resolution genotyping can result in detection of several SNVs such that more than one allele combination is possible. In RHCE, this is common in samples found to be compound heterozygotes for c.48G/C and c.733C/G. These SNVs are known to exist by themselves, together and also with additional SNVs (see Tables 3,4). Table 6 shows the two possible allele assignments in this scenario. In the scenario shown at the top of Table 6, when the SNVs are inherited in cis (on the same allele), the other allele matches the reference. Though the RBCs would be predicted to type V+ and VS+, the patient is not predicted to express partial or variant antigens in the absence of normal antigens. In the scenario shown at the bottom of Table 6, when the SNVs are inherited in trans (on separate alleles), the sample does not carry a reference allele expressing normal antigens. In this case, the individual may be at risk of allo-anti-f since they do not express a normal f antigen. Though standard SNV genotyping panels cannot determine the phase in this scenario, long-range PCR with a sequence-specific primers can be used to determine cis or trans configuration. Additionally, if total RNA can be isolated from erythrocytes, cDNA PCR can be performed to amplify the RHCE transcripts which can be cloned into plasmid vectors such that Sanger sequencing of plasmid DNA isolated from individual bacterial colonies can characterize each allele separately (30). This is depicted in Figure 3 in which Figure 3A depicts the RHCE allele with the SNVs in trans and Figure 3B the alleles with the SNVs in cis. Figure 3C shows the cDNA amplicons from the scenario where the SNVs are in trans and Figure 3D the cDNA amplicons from the scenario where the SNVs are in cis. This case illustrates how the amplification and cloning of RH cDNAs followed by Sanger sequencing can be used to “solve” the phase of a sample carrying multiple SNVs in compound heterozygosity.
Table 6
Possible RHCE allele combinations | Predicted phenotype | Risk of alloimmunization |
---|---|---|
RHCE*01/RHCE*01.20.02.01 (RHCE*ce/RHCE*ce48C,733G) | RH:4 (c+) | None |
RH:5 (e+) | ||
RH:10,20 (V+VS+) | ||
RH:31 (hrB+) | ||
RHCE*01.01/RHCE*01.20.01 (RHCE*ce48C/RHCE*ce733G) | RH:4 (c+) | Allo-anti-f (RH6) |
RH:5 (e+ weak) | Some reports of anti-e-like (possible anti-eVAR) | |
RH:10,20 (V+VS+) | ||
RH:31 (hrB+) |
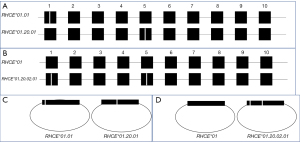
Conclusions
This narrative review describes the limitations of commercially available red cell genotyping panels. The author acknowledges that the cataloguing of the panels was based on experience and internet searches and may be incomplete. In addition, the limitations of genotyping panels were not exhaustive, but instead focused on limitations that may not be well appreciated by clinicians who received genotyping reports or laboratory managers who select and implement the panels in their facilities.
It is important for those performing molecular testing and those utilizing the results of such testing to understand the limitations of current RH genotyping panels, and RBC genotyping panels more generally. However, these limitations should not dissuade clinicians and immunohematology reference laboratory and blood bank staff from utilizing this technology. Serologic methods used to directly test for antigens have limitations as well. Those limitations include lack of reagents, discrepancies due to weak antigens and/or epitope loss in partial antigens especially RhD (31) as well as mixed field reactivity after recent transfusion or in the context of autoantibodies (32).
Though there are limitations to our current understanding of RH genetics and the implications of the inheriting some RH variants, as well as demonstrated efficacy of Rh immune prophylaxis in partial D women, there is much evidence to show that RH genotyping is beneficial in identifying risk of alloimmunization and can also be used to personalized donor unit selection. Currently, low- and medium-resolution testing allows cost-effective and timely resolution of the majority (but not all) cases of serologic weak D phenotypes and typing discrepancies, thus allowing the resulting information to be used for patient management. In complex cases, high resolution methods may be needed that require engaging a molecular reference laboratory that offers such services.
In the future, high-resolution testing is certain to become common place for red cell genotyping including RH genotyping. Although even that technology is not free from challenges with interpreting genetic variation (33,34), molecular methods and software algorithms are improving (35) such that discussions of incorrect phenotype predictions are likely to become a thing of the past. Until that time, it is important for laboratorians performing or ordering RH genotyping as well as clinicians ordering or interpreting RH genotyping to understand the limitations of commercial panels and the value of a molecular reference laboratory with expertise in this area, especially in cases where high-resolution testing is warranted (36).
Acknowledgments
Funding: None.
Footnote
Provenance and Peer Review: This article was commissioned by the Guest Editors (Emilia Sippert, Carine Prisco Arnoni and Maria Rios) for the series “Novel RH Alleles” published in Annals of Blood. The article has undergone external peer review.
Reporting Checklist: The author has completed the Narrative Review reporting checklist. Available at https://aob.amegroups.com/article/view/10.21037/aob-23-18/rc
Peer Review File: Available at https://aob.amegroups.com/article/view/10.21037/aob-23-18/prf
Conflicts of Interest: The author has completed the ICMJE uniform disclosure form (available at https://aob.amegroups.com/article/view/10.21037/aob-23-18/coif). The series “Novel RH Alleles” was commissioned by the editorial office without any funding or sponsorship. M.A.K. reports honoraria from Thomas Jefferson University for lecturing in graduate school course and travel supports from her employer, the American Red Cross, for internal staff meetings and external conferences related to transfusion medicine and molecular immunohematology (SCABB Annual meeting and ISBT Congress). She is the Secretary of South Central Association of Blood (SCABB) and Secretary of International Society of Blood Transfusion (ISBT) Working party for Red Cell Immunogenetics and Blood Group Terminology. The author has no other conflicts of interest to declare.
Ethical Statement: The author is accountable for all aspects of the work in ensuring that questions related to the accuracy or integrity of any part of the work are appropriately investigated and resolved.
Open Access Statement: This is an Open Access article distributed in accordance with the Creative Commons Attribution-NonCommercial-NoDerivs 4.0 International License (CC BY-NC-ND 4.0), which permits the non-commercial replication and distribution of the article with the strict proviso that no changes or edits are made and the original work is properly cited (including links to both the formal publication through the relevant DOI and the license). See: https://creativecommons.org/licenses/by-nc-nd/4.0/.
References
- Red Cell Immunogenetics and Blood Group Terminology. Available online: https://www.isbtweb.org/working-parties/red-cell-immunogenetics-and-blood-group-terminology/
- Reid ME, Yazdanbakhsh K. Molecular insights into blood groups and implications for blood transfusion. Curr Opin Hematol 1998;5:93-102. [Crossref] [PubMed]
- Reid ME, Lomas-Francis C. Molecular approaches to blood group identification. Curr Opin Hematol 2002;9:152-9. [Crossref] [PubMed]
- Reid ME, Denomme GA. DNA-based methods in the immunohematology reference laboratory. Transfus Apher Sci 2011;44:65-72. [Crossref] [PubMed]
- Flegel WA. Molecular genetics of RH and its clinical application. Transfus Clin Biol 2006;13:4-12. [Crossref] [PubMed]
- Reid ME, Halter Hipsky C, Hue-Roye K, et al. Genomic analyses of RH alleles to improve transfusion therapy in patients with sickle cell disease. Blood Cells Mol Dis 2014;52:195-202. [Crossref] [PubMed]
- Chou ST, Evans P, Vege S, et al. RH genotype matching for transfusion support in sickle cell disease. Blood 2018;132:1198-207. [Crossref] [PubMed]
- Fasano RM, Sullivan HC, Bray RA, et al. Genotyping Applications for Transplantation and Transfusion Management: The Emory Experience. Arch Pathol Lab Med 2017;141:329-40. [Crossref] [PubMed]
- Keller MA, Horn T, Crowley J, et al. Genotype compatibility tables for matching patients and donors for Rh variants. Transfusion 2013;53:174A.
- Keller MA, Horn T, Fludd DR, et al. RH Allele Matching for Rh Alloimmunized Patients: Personalized Medicine to the Nth Degree. Transfusion 2019;59:143A.
- Keller MA. The role of red cell genotyping in transfusion medicine. Immunohematology 2015;31:49-52. [Crossref] [PubMed]
- Carritt B, Blunt T, Avent N, et al. Rh null phenotypes are not due to a gross deletion and can occur on different Rh genetic backgrounds. Ann Hum Genet 1993;57:273-9. [Crossref] [PubMed]
- Daniels G. Rh and RHAG blood group systems. In: Human Blood Groups. 3rd ed. Oxford, UK: Blackwell Publishing Ltd; 2013, pp.182-258.
- Floch A. Maternal red blood cell alloimmunisation Working Party, literature review. RH blood group system: Rare specificities. Transfus Clin Biol 2021;28:314-20. [Crossref] [PubMed]
- Pham BN, Peyrard T, Juszczak G, et al. Heterogeneous molecular background of the weak C, VS+, hr B-, Hr B- phenotype in black persons. Transfusion 2009;49:495-504. [Crossref] [PubMed]
- Zhao J, Grant SF. Advances in whole genome sequencing technology. Curr Pharm Biotechnol 2011;12:293-305. [Crossref] [PubMed]
- Montemayor C, Brunker PAR, Keller MA. Banking with precision: transfusion medicine as a potential universal application in clinical genomics. Curr Opin Hematol 2019;26:480-7. [Crossref] [PubMed]
- Westhoff CM, Vege S, Nickle P, et al. Nucleotide deletion in RHCE*cE (907delC) is responsible for a D- - haplotype in Hispanics. Transfusion 2011;51:2142-7. [Crossref] [PubMed]
- Sandler SG, Flegel WA, Westhoff CM, et al. It's time to phase in RHD genotyping for patients with a serologic weak D phenotype. College of American Pathologists Transfusion Medicine Resource Committee Work Group. Transfusion 2015;55:680-9. [Crossref] [PubMed]
- Flegel WA, Denomme GA, Queenan JT, et al. It's time to phase out "serologic weak D phenotype" and resolve D types with RHD genotyping including weak D type 4. Transfusion 2020;60:855-9. [Crossref] [PubMed]
- St-Louis M, Richard M, Côté M, et al. Weak D type 42 cases found in individuals of European descent. Immunohematology 2011;27:20-4. [Crossref] [PubMed]
- Lapadat R, Meyer E, Josephson C, et al. Exon Sequencing Improves Red Blood Cell Phenotype Matching in a patient homozygous for the RHCE*ce48C allele. Transfusion 2015;55:152A.
- Keller MA. RH genetic variation and the impact for typing and personalized transfusion strategies: a narrative review. Ann Blood 2023;8:18. [Crossref]
- Crowley JA, Horn T, Keller MA. RHCE Characterization requires both commercial genotyping arrays and lab-developed tests. Transfusion 2013;53:172A.
- Reference SNP (rs) Report. Available online: https://www.ncbi.nlm.nih.gov/snp/rs57992529
- Meny GM. The Duffy blood group system: a review. Immunohematology 2010;26:51-6. [Crossref] [PubMed]
- Tournamille C, Colin Y, Cartron JP, et al. Disruption of a GATA motif in the Duffy gene promoter abolishes erythroid gene expression in Duffy-negative individuals. Nat Genet 1995;10:224-8. [Crossref] [PubMed]
- Zimmerman PA, Woolley I, Masinde GL, et al. Emergence of FY*A(null) in a Plasmodium vivax-endemic region of Papua New Guinea. Proc Natl Acad Sci U S A 1999;96:13973-7. [Crossref] [PubMed]
- Arndt PA, Horn T, Keller JA, et al. First example of an FY*01 allele associated with weakened expression of Fya on red blood cells. Immunohematology 2015;31:103-7. [Crossref] [PubMed]
- Smith A, Lindquist M, Williams ML, et al. Donor C or E Typing Discrepancies Lead to Identification of Novel RHCE Alleles. Transfusion 2022;62:130A.
- Horn TN, Keller J, Keller MA, et al. Identifying obstetrics patients in whom RHD genotyping can be used to assess risk of D alloimmunization. Immunohematology 2020;36:146-51. [Crossref] [PubMed]
- Horn T, Hamilton J, Kosanke J, et al. Assessment of common red blood cell pretreatments to yield an accurate serologic antigen phenotype compared with genotype-predicted phenotype. Immunohematology 2019;33:147-51. [Crossref] [PubMed]
- Montemayor C, Brunker PAR, Keller MA. Banking with precision: transfusion medicine as a potential universal application in clinical genomics. Curr Opin Hematol 2019;26:480-7. [Crossref] [PubMed]
- Halls JBL, Vege S, Simmons DP, et al. Overcoming the challenges of interpreting complex and uncommon RH alleles from whole genomes. Vox Sang 2020;115:790-801. [Crossref] [PubMed]
- Zhang Z, An HH, Vege S, et al. Accurate long-read sequencing allows assembly of the duplicated RHD and RHCE genes harboring variants relevant to blood transfusion. Am J Hum Genet 2022;109:180-91. [Crossref] [PubMed]
- Sandler SG, Horn T, Keller J, et al. A model for integrating molecular-based testing in transfusion services. Blood Transfus 2016;14:566-72. [PubMed]
Cite this article as: Keller MA. Challenges with assigning RH alleles and accurately predicting phenotypes using commercially-available genotyping kits: a narrative review. Ann Blood 2024;9:4.