Implication of novel RH alleles in blood transfusion therapy
Introduction
Background
The Rh blood group system is among the most immunogenic and polymorphic blood group and consists of 56 antigens encoded by two homologous genes located on chromosome 1, RHD and RHCE. Most antigens are encoded by the RHCE gene and a small number are encoded by RHD or hybrid RHCE with RHD. The most common antigens are D (RH1), C (RH2), c (RH4), E (RH3) and e (RH5) detected by commercial reagent antibodies. There are over 400 RHD and over 150 RHCE alleles recognized by International Society of Blood Transfusion (ISBT) (1), but many more alleles can be found in other database inventories as the RhesusBase (2), RHeference database (3), Erythrogene (4). Those alleles are result of single nucleotide variants, short/large insertions/deletions, gene rearrangements and gene deletion (5). This level of complexity can result in altered expression of D and CE antigens on red blood cells (RBCs) with critical transfusion outcomes and high rates of alloimmunization. Clinical or biological evidence of these effects are not available for all RH variant alleles as not all Rh antigens have been associated with antibody formation and not all alloantibodies produced are clinically significant (6,7).
Rationale and knowledge gap
There are 2 types of Rh variants, the weak Rh antigens that conceptually do not lack immunogenic epitopes and have weak expression and the partial Rh antigens that conceptually lack immunogenic epitopes and have weak or normal expression (8,9). Historically, weak antigens do not induce alloimmunization and partial antigens induce alloimmunization and are considered clinically significant, as the carrier of partial antigens exposed to a complete antigen can produce antibodies against the missing epitopes and against high-frequency antigens such as RH31 (hrB) and RH19 (hrS) (10-12). However, the limit of these two concepts is not simple as demonstrated by Daniels et al. (13), since it is not possible to predict that all patients with weak antigens will not produce antibodies and that all patients with partial antigens are at risk of antibody formation.
Although the clinical significance of Rh variants is not easy to establish, RH genotyping has been recommended to resolve serologic weak phenotypes or discrepancies found in the routine and to differentiate weak and partial Rh antigens (8,10-16). However in recent years, an increasing number of novel RH alleles have been discovered but their serological descriptions, antibody formation risk and implication in blood transfusion have still been difficult to demonstrate as many of these alleles have only been observed once in a donor or in a non-alloimmunized patient. In addition, the variant type, allelic forms, and trimeric conformation of Rh proteins influence the clinical significance of antibodies. In many cases, functional analysis and intraprotein interactions have been used to characterize the effect of the variations at the molecular level (17-20).
Objective
A better understanding of alloimmunization risk and clinical significance of the antibodies produced by these novel RH alleles are necessary to improve transfusion safety.
RH alleles and blood transfusion therapy
RHD alleles and risk of anti-D
It is important to be aware that not all carriers of variant RHD alleles will develop anti-D. The distinction between RHD alleles encoding weak D and partial D antigens can be of clinical importance to predict the risk of alloimmunization and their implication in blood transfusion therapy. There are over 700 RHD alleles registered in the RHeference database but only 30% of them have been associated with anti-D formation (3). In a narrative review of RH alleles and antibodies, Floch (21) reported the most common RHD alleles associated with production of anti-D according to the literature. Most are RHD alleles encoding partial D but weak D types 11, 15, 21, 41, 42 and 45 have also been associated with anti-D formation. Due to the lack of evidence on alloimmunization in carriers of the 5 most prevalent weak D types 1, 2, 3, 4.0, 4.1, it has been recommended that they should be treated as D-positive to conserve the limited supply of D-negative blood while the less common types including weak D type 4.2 that is commonly found in Africans should be treated as D-negative (22). This is one of the reasons that RHD genotyping is being recommended to differentiate the weak D types and partial D antigens in transfused patients (8,16,23-25). In a recent study, Miranda et al. (11) performing systematic RHD genotyping in transfused patients serologically typed as weak D receiving D-positive RBC units showed that 63.2% of them had RHD alleles encoding partial D and therefore should be treated as D-negative. The prevalence of novel alleles can vary significantly among different populations and there are still rare reports on alloanti-D immunization of these sporadic RHD variant alleles. Therefore, in the absence of clinical information about anti-D formation patients carrying these rare RHD alleles have been treated as D-negative (21). Testing RBCs with different anti-D clones can provide potential insight into D epitope profile as the absence of epitopes suggests that these alleles encode partial D phenotypes with potential risk of D formation (26,27). Bioinformatics studies have also revealed the effects of amino acid substitutions on interactions within the RhD molecule and can be helpful in assessing new variants (20,28). The type of nucleotide variation also provides information, for example, changes that occur at an exon splicing site are predicted to result in a reduced level of D transcripts responsible for weak D typing, but not an amino acid change, and therefore these alleles would not be predicted to be associated with alloimmunization (29). Prospective studies on the anti-D formation risk of novel RHD alleles, especially those reported in a large number of carriers are necessary to understand their clinical significance. D epitope expression of the novel RHD alleles determined by serological tests and expression studies in vitro would also be helpful. Without these studies it seems premature to exclude their implication in blood transfusion. In addition, there are some alleles encoding antigens with a very low antigen density which tend to type as D-negative and therefore the carriers are less likely to receive D-positive RBC units (30). There are also some intriguing situations regarding the production of antibodies by RHD alleles encoding variant antigens as for example the individuals with a compound heterozygous variant RHD alleles. Even in the presence of an allele encoding a partial antigen, depending on the other allele, the association with RHCE allelic forms and the trimeric conformation of Rh proteins, the risk of anti-D formation may vary (10,12,23). Identifying compound heterozygous individuals can be clinically relevant because patients with weak D types 1, 2, 3, 4.0, or 4.1 will not need to be transfused with D− RBCs (22). Novel RHD alleles often produce discrepant or inconclusive serological and molecular results but the causes for such discrepancies should be identified for the benefit of patient care and the advancement of allele databases. The bioinformatics analysis of RhD protein can help us understand the impact of RHD variants on antigen differences and guide blood transfusion strategies. The impact of novel RHD molecular variations located in splicing regions has also been investigated using a functional test, namely minigene splicing assay that could be another tool for interpretating the functional effect and/or related phenotype of novel RHD alleles to provide more precision transfusion guidance (17,31,32). The decision to treat a patient as D+ or D− should consider, in addition to the specific allele, the patient age, gender, history of antibody production, transfusion needs and the blood supply.
Implication of RHCE variant alleles in transfusion
The genetic diversity of the RH locus in populations of African origin contributes to the high prevalence of altered RHCE alleles and with high rate of alloimmunization in sickle cell disease (SCD) patients (10,33). In addition to variant RHCE alleles predicting partial antigens there are many alleles that also predict the loss of high prevalence antigens such as hrB (RH31) and hrS (RH19) that should be taken into account in the selection of RBC units for transfusion (34). Anti-e is the most frequent alloantibody observed in SCD patients with variant RHCE alleles, although not all are implicated in hemolytic transfusion reactions (HTRs) suggesting that the alloimmunization risk and the clinical significance of Rh antibodies produced may vary according to the specific variant inherited (21). In a recent study, we observed that most of patients with the RHCE*01.20.01 (RHCE*ce733G) allele who developed anti-e had a good survival of the transfused RBCs when exposed to conventional e antigen while patients with the RHCE*01.04.01 (RHCE*ceAR) allele produced clinically significant anti-e and anti-hrS (34). Indeed, there is evidence that some RHCE alleles such as RHCE*01.01 (RHCE*ce48C), RHCE*01.20.01 (RHCE*ce733G) and RHCE*01.20.02 (RHCE*ce 48C,733G) have a low risk for antibody formation or implication in severe hemolytic episodes (6,10,34). In addition, it has been suggested that the presence of one conventional RH allele is not protective against alloimmunization (10,35,36). RHCE alleles previously associated with antibody formation to the corresponding antigens were reported with RH10 (V) and RH20 (VS) phenotypes (10,21) and no antibody formation was associated with rare RHCE alleles, such as, RHCE*02.02, RHCE*02.03 and RHCE*02.11 with no RH10 or RH20 phenotypes (37-39). Although extremely important, the clinical significance of RHCE variant alleles is not easy to establish in polytransfused patients, especially whether the antibody formed is an alloantibody or autoantibody as auto-adsorptions are not recommended for recently transfused patients (40). Moreover, autoantibodies can cause hemolysis and therefore the antibody implication in an HTR may be difficult to ascertain. The difficulty in finding donors with well-characterized RHCE alleles to select units based on patient’s RH alleles, associated with little knowledge of the risk of alloimmunization and the clinical significance of the antibody, makes the transfusion decision difficult (41). In order to understand the implication of the novel RHCE alleles in transfusion therapy, it is important to know their frequency in the population studied and demonstrate that the allele predicts an antigen at risk for antibody formation and a loss of the high prevalence antigens hrB (RH31) and hrS (RH19) (6,21,36). It is important to emphasize that the selection of compatible blood for a patient with a rare allele also depends on the availability of genotyped donors for RH variant alleles as this request is filled with RH genotype-selected units.
Novel RH alleles, alloimmunization and hemolytic risks
High resolution genotyping has revealed a plethora of novel RH alleles in different populations as a result of serological discrepancies or discordances between serologic typing and genotype-predicted phenotype (36,42-44). However, many alleles have been reported in abstract form, have no prevalence associated, and few of the novel alleles reported to-date were associated with production of antibody to the expressed antigen. Most of RH novel alleles have been described in donors or in non-alloimmunized patients and in many of these studies, even when an antibody is produced, the serology is not well detailed, mainly in the discrimination of auto and alloantibodies. The risk of alloimmunization is often associated with the type of variant Rh antigen that the new allele encodes, that is, a weak antigen or a partial antigen, however, more serological, and clinical studies would be valuable to determine the risk for antibody formation. It is difficult to measure the risk of alloimmunization of novel alleles, since most patients carrying partial Rh antigens are not routinely recognized unless they form unexpected antibodies. Furthermore, the Rh antibodies formation may be influenced by personal characteristics, since it is known that some individuals are less likely to produce alloantibodies and are “low responders” despite multiple transfusions (45). There are some RH alleles widely accepted for producing partial antigens and are associated with the formation of alloantibodies if exposed to a conventional antigen as for example the (C)ceS haplotype but even so it varies in different populations (10,12,34,35). Therefore, it is very difficult to establish a strategy to reduce the risk of alloimmunization if we do not properly know the antigen characteristic. In general, new alleles have emerged with advances in molecular techniques with very little information about the antigens they encode or the formation of antibodies. Furthermore, a study observed that altered epitopes on donor red cells can also induce alloimmunization and this is very difficult to control (46). In the discovery of novel RH alleles, it is very important to provide serological data, even if incomplete, alloimmunization information and clinical data so that we can assess the implication of that allele in blood transfusion therapy and propose a strategy to prevent alloimmunization and HTRs (47). In a study performed by Coleman et al. (35) on alloimmunization and implication of antibodies produced with delayed hemolytic transfusion reactions (DHTRs) they observed that 47% of clinically significant Rh antibodies occurred in patients with at least one allele encoding any partial Rh antigen. Although phenotype and clinical data are the standard determinants for assessing the effect of genetic variations, the use of bioinformatics prediction and modeling tools have evolved and currently studies of 3D intraprotein interactions combined with epitope mapping and antigen density have been used in assessing the risk of alloimmunization in carriers of RH variants (19,20). An investigation of intraprotein interactions of the product of 25 RHD variant alleles on the risk of anti-D alloimmunization performed by Floch et al. (19) showed that extracellular changes and the alteration of intraprotein interactions were correlated with the risk of anti-D formation in 76% of variants with anti-D. Currently, functional and clinical interpretation of variations in blood group genes by 3D-protein structure investigation have been considered an additional tool for assessing the risk of antibody formation in patients carrying novel variant RH and may become valuable for guiding the implication of novel alleles in hemolytic risks, especially when no serological or clinical data are available. In silico processed bioinformatics analysis may also improve our understanding of the influence of genetic variants on antigen expression and its implications for blood transfusion therapy (48-50). Figure 1 illustrates a case of a patient with SCD typed as D-positive with anti-D in his serum on how 3D analysis could be used when new RH variants are detected (46). In this particular sample, the authors attempted to take advantage of combining the molecular data and available silico tools for the functional interpretation of the new variant, in order to get insights into the clinical phenotype that may be predicted a priori from genotyping.
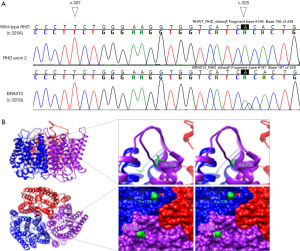
Conclusions
It’s worth noting that medical advancements and improved understanding of the RH system have led to more accurate testing and screening procedures. High-resolution RH typing is beginning to be performed using advanced sequencing technologies with increased average read length that allows identification of structural variants, sequencing repetitive regions, phasing of distant nucleotide changes, and distinguishing highly homologous genomic regions, thereby improving the characterization of RHD and RHCE genes, and increasing the discovery of variants relevant to blood transfusion. Immunization potential of novel RHD and RHCE alleles is difficult to predict. For now, the recommendation is that patients be treated as antigen negative in transfusion, until evidence emerges that can prove the risk or not of antibody formation. In the pregnancy management, without knowledge of the potential risk of alloimmunization, all women with a novel RHD allele will be considered D− and will receive comprehensive monitoring, including additional antibody detection test, RHD fetal genotyping and RhIG injection. Hopefully, more serological, epidemiological, and clinical data with the incidence and severity of DHTRs associated with antibody formation, particularly if documented for larger cohorts, will be reported with the novel alleles being discovered so that the transfusion recommendation and pregnant management will be more accurate. A better understanding of the involvement of novel RH alleles with the production of antibodies and with a potential risk of causing HTRs may refine RH molecular matching and increase transfusion safety. The bioinformatics of these alleles can extend our knowledge of Rh variants and help assess their implications in blood transfusion therapy. For the past years, due to the advent of next-generation sequencing and the subsequent identification of numerous rare variants, bioinformatics prediction and modelling tools have evolved and currently help physicians in diagnostics, clinical management and genetic counselling. Although phenotype and clinical symptoms remain definitely the standard determinants to assess the effect of genetic variations, use of those approaches may soon become valuable for guiding subsequent investigations in immunohematology.
Overall, the discovery and understanding of novel RH alleles contribute to the improvement of blood transfusion practices. They help enhance donor-recipient compatibility, refine screening and testing procedures, and ensure the safety of transfused blood products. Regular updates and advancements in blood bank protocols are crucial to accommodate the evolving knowledge of RH alleles and reduce the risk of alloimmunization. Accurate determination of RhD status, identification of RhCE variants, and monitoring for alloimmunization risk are essential in providing safe and effective transfusion therapy to patients. Molecular techniques play a crucial role in identifying novel RH alleles and improving transfusion practices.
Acknowledgments
The author would like to thank Dr. Yann Fichou from the Etablissement Français du Sang - Bretagne, France for his help on the functional and clinical interpretation of variations in RH genes by 3D-protein structure investigation and Dr. Simone Gilli from Hemocentro Unicamp, Campinas, Brazil for fruitful discussions on the clinical significance of variant RH alleles.
Funding: This study was supported by Fundação de Amparo à Pesquisa do Estado de São Paulo (FAPESP) (No. 2020/02191-1).
Footnote
Provenance and Peer Review: This article was commissioned by the Guest Editors (Emilia Sippert, Carine Prisco Arnoni and Maria Rios) for the series “Novel RH Alleles” published in Annals of Blood. The article has undergone external peer review.
Peer Review File: Available at https://aob.amegroups.com/article/view/10.21037/aob-23-26/prf
Conflicts of Interest: The author has completed the ICMJE uniform disclosure form (available at https://aob.amegroups.com/article/view/10.21037/aob-23-26/coif). The series “Novel RH Alleles” was commissioned by the editorial office without any funding or sponsorship. The author has no other conflicts of interest to declare.
Ethical Statement:
Open Access Statement: This is an Open Access article distributed in accordance with the Creative Commons Attribution-NonCommercial-NoDerivs 4.0 International License (CC BY-NC-ND 4.0), which permits the non-commercial replication and distribution of the article with the strict proviso that no changes or edits are made and the original work is properly cited (including links to both the formal publication through the relevant DOI and the license). See: https://creativecommons.org/licenses/by-nc-nd/4.0/.
References
- Available online: https://www.isbtweb.org/isbt-working-parties/rcibgt.html
- Available online: http://www.rhesusbase.info/introduceRhesusBase.htm
- Available online: https://www.rheference.org/
- Available online: http://www.erythrogene.com/
- Flegel WA. Molecular genetics and clinical applications for RH. Transfus Apher Sci 2011;44:81-91. [Crossref] [PubMed]
- Noizat-Pirenne F, Tournamille C. Relevance of RH variants in transfusion of sickle cell patients. Transfus Clin Biol 2011;18:527-35. [Crossref] [PubMed]
- Silvy M, Tournamille C, Babinet J, et al. Red blood cell immunization in sickle cell disease: evidence of a large responder group and a low rate of anti-Rh linked to partial Rh phenotype. Haematologica 2014;99:e115-7. [Crossref] [PubMed]
- Daniels G. Variants of RhD--current testing and clinical consequences. Br J Haematol 2013;161:461-70. [Crossref] [PubMed]
- Wagner FF, Gassner C, Müller TH, et al. Molecular basis of weak D phenotypes. Blood 1999;93:385-93. [Crossref] [PubMed]
- Chou ST, Jackson T, Vege S, et al. High prevalence of red blood cell alloimmunization in sickle cell disease despite transfusion from Rh-matched minority donors. Blood 2013;122:1062-71. [Crossref] [PubMed]
- Miranda MR, Dos Santos TD, Castilho L. Systematic RHD genotyping in Brazilians reveals a high frequency of partial D in transfused patients serologically typed as weak D. Transfus Apher Sci 2021;60:103235. [Crossref] [PubMed]
- Sippert E, Fujita CR, Machado D, et al. Variant RH alleles and Rh immunisation in patients with sickle cell disease. Blood Transfus 2015;13:72-7. [PubMed]
- Daniels G, Poole G, Poole J. Partial D and weak D: can they be distinguished? Transfus Med 2007;17:145-6. [Crossref] [PubMed]
- Noizat-Pirenne F, Lee K, Pennec PY, et al. Rare RHCE phenotypes in black individuals of Afro-Caribbean origin: identification and transfusion safety. Blood 2002;100:4223-31. [Crossref] [PubMed]
- Westhoff CM. Blood group genotyping. Blood 2019;133:1814-20. [Crossref] [PubMed]
- Sandler SG, Flegel WA, Westhoff CM, et al. It's time to phase in RHD genotyping for patients with a serologic weak D phenotype. College of American Pathologists Transfusion Medicine Resource Committee Work Group. Transfusion 2015;55:680-9. [Crossref] [PubMed]
- Raud L, Ka C, Gourlaouen I, Callebaut I, et al. Functional analysis of novel RHD variants: splicing disruption is likely to be a common mechanism of variant D phenotype. Transfusion 2019;59:1367-75. [Crossref] [PubMed]
- Conroy MJ, Bullough PA, Merrick M, et al. Modelling the human rhesus proteins: implications for structure and function. Br J Haematol 2005;131:543-51. [Crossref] [PubMed]
- Floch A, Pirenne F, Barrault A, et al. Insights into anti-D formation in carriers of RhD variants through studies of 3D intraprotein interactions. Transfusion 2021;61:1286-301. [Crossref] [PubMed]
- de Brevern AG, Floch A, Barrault A, et al. Alloimmunization risk associated with amino acid 223 substitution in the RhD protein: analysis in the light of molecular modeling. Transfusion 2018;58:2683-92. [Crossref] [PubMed]
- Floch A. Molecular genetics of the Rh blood group system: alleles and antibodies—a narrative review. Ann Blood 2021;6:29. [Crossref]
- Flegel WA, Denomme GA, Queenan JT, et al. It's time to phase out "serologic weak D phenotype" and resolve D types with RHD genotyping including weak D type 4. Transfusion 2020;60:855-9. [Crossref] [PubMed]
- Westhoff CM. Rh complexities: serology and DNA genotyping. Transfusion 2007;47:17S-22S. [Crossref] [PubMed]
- Clarke G, Hannon J, Berardi P, et al. Resolving variable maternal D typing using serology and genotyping in selected prenatal patients. Transfusion 2016;56:2980-5. [Crossref] [PubMed]
- Floch A, Barrault A, De Brevern AG, et al. Molecular characterization of 13 new RHD alleles. Transfusion 2017;57:1089-91. [Crossref] [PubMed]
- Jones J, Scott ML, Voak D. Monoclonal anti-D specificity and Rh D structure: criteria for selection of monoclonal anti-D reagents for routine typing of patients and donors. Transfus Med 1995;5:171-84. [Crossref] [PubMed]
- Wagner FF, Gassner C, Muller TH, et al. Three molecular structures cause rhesus D category VI phenotypes with distinct immunohematologic features. Blood 1998;91:2157-68. [Crossref] [PubMed]
- Zhang X, Li G, Zhou Z, et al. Molecular and computational analysis of 45 samples with a serologic weak D phenotype detected among 132,479 blood donors in northeast China. J Transl Med 2019;17:393. [Crossref] [PubMed]
- Chun S, Yun JW, Park G, et al. The synonymous nucleotide substitution RHD 1056C>G alters mRNA splicing associated with serologically weak D phenotype. J Clin Lab Anal 2018;32:e22330. [Crossref] [PubMed]
- Mota M, Fonseca NL, Rodrigues A, et al. Anti-D alloimmunization by weak D type 1 red blood cells with a very low antigen density. Vox Sang 2005;88:130-5. [Crossref] [PubMed]
- Fichou Y, Gehannin P, Corre M, et al. Extensive functional analyses of RHD splice site variants: Insights into the potential role of splicing in the physiology of Rh. Transfusion 2015;55:1432-43. [Crossref] [PubMed]
- Raud L, Le Tertre M, Vigneron L, et al. Missense RHD single nucleotide variants induce weakened D antigen expression by altering splicing and/or protein expression. Transfusion 2021;61:2468-76. [Crossref] [PubMed]
- Chou ST, Westhoff CM. Molecular biology of the Rh system: clinical considerations for transfusion in sickle cell disease. Hematology Am Soc Hematol Educ Program 2009;178-84. [Crossref] [PubMed]
- Arnoni CP, Vendrame TAP, Silva FS, et al. RHCE variant alleles and risk of alloimmunization in Brazilians. Immunohematology 2022;38:123-9. [PubMed]
- Coleman S, Westhoff CM, Friedman DF, et al. Alloimmunization in patients with sickle cell disease and underrecognition of accompanying delayed hemolytic transfusion reactions. Transfusion 2019;59:2282-91. [Crossref] [PubMed]
- Chou ST, Flanagan JM, Vege S, et al. Whole-exome sequencing for RH genotyping and alloimmunization risk in children with sickle cell anemia. Blood Adv 2017;1:1414-22. [Crossref] [PubMed]
- Bugert P, Scharberg EA, Geisen C, et al. RhCE protein variants in Southwestern Germany detected by serologic routine testing. Transfusion 2009;49:1793-802. [Crossref] [PubMed]
- Scharberg EA, Green C, Daniels G, et al. Molecular basis of the JAHK (RH53) antigen. Transfusion 2005;45:1314-8. [Crossref] [PubMed]
- Vrignaud C, Ramelet S, Joffrin C, et al. CP239: RHCE*Ce286A Is a Novel RHCE Allele That Causes a Weak C Expresion and Codes for the Low-Prevalence LOCR (RH55) Antigen. Transfusion 2017;57:156A.
- Gaspardi AC, Sippert EA, De Macedo MD, et al. Clinically relevant RHD-CE genotypes in patients with sickle cell disease and in African Brazilian donors. Blood Transfus 2016;14:449-54. [PubMed]
- Santos TD, Macedo MD, Menegati SFP, et al. Challenges in providing compatible blood with Rh genotype-matching in Brazilian patients with sickle cell disease. Transfus Med 2019;29:423-9. [Crossref] [PubMed]
- Wheeler MM, Lannert KW, Huston H, et al. Genomic characterization of the RH locus detects complex and novel structural variation in multi-ethnic cohorts. Genet Med 2019;21:477-86. [Crossref] [PubMed]
- Zhang Z, An HH, Vege S, et al. Accurate long-read sequencing allows assembly of the duplicated RHD and RHCE genes harboring variants relevant to blood transfusion. Am J Hum Genet 2022;109:180-91. [Crossref] [PubMed]
- Chang TC, Haupfear KM, Yu J, et al. A novel algorithm comprehensively characterizes human RH genes using whole-genome sequencing data. Blood Adv 2020;4:4347-57. [Crossref] [PubMed]
- Yazdanbakhsh K, Ware RE, Noizat-Pirenne F. Red blood cell alloimmunization in sickle cell disease: pathophysiology, risk factors, and transfusion management. Blood 2012;120:528-37. [Crossref] [PubMed]
- Macedo MD, Miranda MR, Santos TD, et al. Rh antibodies as a result of altered Rh epitopes on transfused red cells: a case series of seven Brazilian patients. Blood Transfus 2021;19:413-9. [PubMed]
- Keller MA. RH genetic variation and the impact for typing and personalized transfusion strategies: a narrative review. Ann Blood 2023;8:18. [Crossref]
- Castilho L, Arnoni C, Vendrame T, et al. From genotyping to the functional and clinical interpretation of variations in blood group genes by 3D-protein structure investigation: two novel variant alleles in the RHD gene. Vox Sang 2019;114:185.
- Ying Y, Zhang J, Hong X, et al. The Significance of RHD Genotyping and Characteristic Analysis in Chinese RhD Variant Individuals. Front Immunol 2021;12:755661. [Crossref] [PubMed]
- Floch A, Vege S, Cai C, et al. Serologic and molecular investigation of five samples uncovers four novel RHD alleles. Transfusion 2022;62:20A.
Cite this article as: Castilho L. Implication of novel RH alleles in blood transfusion therapy. Ann Blood 2023;8:38.