A narrative review of transplant-associated thrombotic microangiopathy: pathogenesis and novel therapies
Introduction
Background
Transplant-associated thrombotic microangiopathy (TA-TMA) is a life-threatening complication after stem cell transplantation. However, delayed diagnosis occurs due to the similarity in clinical presentation to other post-transplant complications in the early stages of development. Transplantation-associated factors contribute to the disturbance of the oxidative microenvironment, complement dysregulation, endothelial injury, and microvascular hemolytic anemia, which can lead to multi-organ failure in patients, and its morbidity and mortality after transplantation are high (1-3). TA-TMA manifests as thrombotic microangiopathy: thrombocytopenia, microangiopathic hemolytic anemia, and organ dysfunction. Clinical symptoms of TA-TMA include renal insufficiency, serositis, hypertension, pulmonary hypertension, diffuse alveolar hemorrhage, posterior reversible encephalopathy syndrome, and gastrointestinal bleeding (4,5).
The gold standard for TA-TMA diagnosis is the pathological diagnosis, i.e., microscopic visualization of significant microvascular thrombosis. However, this is not generally recommended due to the risk of bleeding and infection in post-transplant patient biopsies. The diagnosis of TA-TMA currently relies instead on clinical diagnosis. Of the multiple criteria for TA-TMA, at least four of the following seven features must be met: thrombocytopenia, hemolytic anemia, schistocytes on a peripheral blood smear, elevated lactate dehydrogenase (LDH), proteinuria of at least 30 mg/dL in random urine, hypertension, and high soluble membrane attack complex (MAC) (sC5b-9) (6,7). A clinical diagnosis is needed to anticipate TA-TMA before developing multi-organ dysfunction, as other post-transplant complications can present similarly. And due to the lack of a consistent definition of the clinical diagnosis of TA-TMA, the incidence and prognosis of the syndrome are difficult to estimate and quantify (8-11). However, what is clear is that TA-TMA has an apparent detrimental effect on overall survival (OS) and non-recurrent mortality (NRM) (8,10,11). We present this article in accordance with the Narrative Review reporting checklist (available at https://aob.amegroups.com/article/view/10.21037/aob-22-22/rc).
Rational and knowledge gap
The pathogenesis of TA-TMA is a combination of factors that lead to microvascular endothelial cell damage, which in turn causes platelet aggregation and eventually leads to massive microthrombosis and organ failure in patients (4). However, the specific pathogenesis is still unclear. Multiple therapeutic approaches targeting the pathogenesis have been explored but with different efficacy and prognosis. The most promising targeted therapy is complemented blockade. Terminal complement blockade such as eculizumab has become a well-recognized treatment (12-15). Narsoplimab (OMS721), another complement blocker, has recently received a priority review by the Food and Drug Administration (FDA) (16). Current research on TA-TMA is still focused on abnormal complement activation and related treatment, and previous reviews have mainly summarized these related contents (17,18), while the effects of inflammation and oxidative microenvironment on TA-TMA are rarely summarized.
Objective
This review adds the effects of inflammation and oxidative microenvironment on TA-TMA and the possible treatment options, hoping to help in the clinical treatment of TA-TMA.
Methods
We collected published literature related to TA-TMA up to August 2022 to summarize the pathogenesis, treatment, and outlook of TA-TMA, combined with our own studies related to TA-TMA. The specific flow is shown in Table 1.
Table 1
Items | Specification |
---|---|
Date of search | 2022-08-14 |
Databases and other sources searched | PubMed, Embase |
Search terms used | ((((“Thrombotic Microangiopathies”[Mesh]) OR (Microangiopathies, Thrombotic [Title/Abstract])) OR (Microangiopathy, Thrombotic[Title/Abstract])) OR (Thrombotic Microangiopathy[Title/Abstract])) AND ((((“Stem Cell Transplantation”[Mesh]) OR (Stem Cell Transplantations[Title/Abstract])) OR (Transplantations, Stem Cell[Title/Abstract])) OR (Transplantation, Stem Cell[Title/Abstract])) |
Timeframe | 1990.8–2022.8 |
Inclusion and exclusion criteria | Literature that is consistent with the TA-TMA topic of interest is used as a reference. TTP and other diseases were excluded. Article type included original manuscripts, reviews, and case reports. Language was limited in English |
Selection process | There were two independent authors who performed the search and merge separately |
TA-TMA, transplant-associated thrombotic microangiopathy; TTP, thrombotic thrombocytopenic purpura.
Pathogenesis
Overview
TA-TMA is a clinical syndrome characterized by endothelial injuries like hemolytic uremic syndrome (HUS) and thrombotic thrombocytopenic purpura (TTP) (19). It is characterized by a disturbed inflammatory-oxidative microenvironment, complement activation, and endothelial injury (6), rather than absolute or relative deficiency of ADAMTS13 (20). Various factors during transplantation lead to complement activation, endothelial injury, and microvascular thrombosis, ultimately leading to end-organ dysfunction. Dvorak et al. put forward a “three-hit” hypothesis for TA-TMA pathogenesis: an underlying propensity for complement activation or endothelial damage (first hit), a toxic conditioning regimen leading to endothelial damage (second hit), drugs, alloreactivity, and infection-caused damage (third hit). The combination of the three hits exceeds the threshold that triggers activation of the complement cascade and micro thrombosis (5). Multiple causes before and after transplantation can increase procoagulant factors, soluble adhesion molecules, and pro-inflammatory cytokines, further promoting endothelial damage and initiating complement activation-related pathways (21,22). Nitric oxide inhibits microvascular dilation, reduces P-selectin release from von Willebrand factor (vWF), and gives rise to platelet aggregation and microthrombus formation (23,24). These findings are not only present in microthrombi but also other types of thrombi. The pathogenesis of TA-TMA seems to be highly related to hosting factors. Previous studies have confirmed the role of complement activation, as displayed by the significant increase in sC5b-9 and C3b (6,25). Our previous study showed that TA-TMA patients had elevated levels of complement sC5b-9 and CH50 compared to veno-occlusive disease (VOD), infection, and graft-versus-host disease (GVHD), with implications for identifying post-transplant complications (25).
In contrast, patients with GVHD and TA-TMA have elevated C3b, suggesting TA-TMA in combination with GVHD (25). In addition, the time of onset and the duration of GVHD correlated with the start of TA-TMA (26). During transplantation, recipients can acquire complement system regulatory protein variants from the donor, which increases the risk of developing TA-TMA, suggesting that both donors and recipients should be screened for relevant genes before hematopoietic stem cell transplantation (HSCT) to select a better donor (16). HLA-DRB1*11 is strongly related to the development of idiopathic TTP (27,28). In TA-TMA patients, HLA-DRB1*11 patients have a better prognosis than average controls (29).
Complement system
According to several previous studies, patients with TA-TMA often present with dysregulation of the complement system. Several pieces of evidence have confirmed the role of abnormal complement activation in the pathogenesis of other thrombotic microangiopathies such as atypical hemolytic uremic syndrome (aHUS). Defects in regulatory proteins associated with the complement system’s alternative pathway in patients with aHUS lead to a large production of the C3-converting enzyme C3bBb. This convertase can form additional C3b molecules, the activated form of C3, leading to the accumulation of the C3bBb-C3b, which ultimately promotes the generation of the cellular C5b-9 MAC, causing endothelial cell damage and ultimately TA-TMA. aHUS defects in complement regulation can be determined by the production of neutralizing autoantibodies against factor H, thereby promoting abnormal complement activation (30).
In a study by Jodele et al. involving six patients with TA-TMA, these patients were found to have defective complement regulation (31). These patients displayed a high rate of CFHR3-CFHR1 heterozygous deletion (83%) compared to only 33% found in a control population, which is close to 25% in general population (32). And in these six patients, three also exhibited the presence of factor H autoantibodies. The CFHR1 haploinsufficiency that leads to partial deletion may lead to dysregulation of C5 convertase activity. At the same time, autoantibodies that neutralize factor H may also lead to uninhibited formation of the cell surface alternative pathway C3 convertase. Most patients (67%) responded well to antibody clearance therapies such as rituximab and therapeutic plasma exchange (TPE) (32).
Although activation due to abnormalities in the complement system and microvascular endothelial damage are the primary mechanisms contributing to the pathogenesis of aHUS, emerging evidence from clinical and basic experiments suggests a direct role for complement in promoting other types of TMA. Patients with complement TTP have severe ADAMTS13 defects, and their vWF multimers similarly activate complement, and mouse models of TTP again show the presence of abnormal complement activation (33). In addition, patients with refractory relapsed TTP had a higher frequency of complement abnormalities, and elevated levels of plasma C5a, C3a, and sC5b-9 were also detected, and patients’ prognosis was improved with plasmapheresis (34,35).
Previous studies have confirmed that Shiga toxin disrupts the biological activity of factor H, which results in diminished inhibition of C3, leading to elevated levels of sC5b-9 and abnormal complement activation in patients with Shiga toxin-associated hemolytic uremic syndrome (STEC-HUS) (36,37). Based on these studies, STEC-HUS patients were successfully treated with eculizumab, which was essential in the 2011 STEC-HUS outbreak in Germany (38,39). Some researchers have even speculated that all TMA is a complement-mediated disease (40). The complement disorder also seems to be involved in developing “secondary” TMA, such as HELLP (Hemolysis, Elevated Liver enzymes and Low Platelets) syndrome, systemic lupus erythematosus, and malignant hypertension (41-44). The HSCT status and the abnormal activation of complement are among the features that distinguish TA-TMA from other TMAs.
Oxidation microenvironment
Redox is a necessary life process of the organism. Some investigations have shown that oxidative stress can induce the formation of thrombosis (45,46). Heme oxygenase-1 (HO-1) is a regulatory protein mediated by oxidative stress that protects cell membranes by degrading heme to ferrous iron, carbon monoxide, and biliverdin (47). In response to oxidative stress, it protects vascular endothelial cells from various factors (48,49). HO-1 attenuates cellular damage through its anti-apoptotic, antioxidant, and anti-inflammatory effects (50,51). Dysfunctions of HO-1 are observed in multiple diseases, including vascular injury (52), hypoxic lung disease (53), and cardiac transplant rejection (54), especially acute myocardial infarction (55). What’s more, it prevents abnormal complement activation by stimulating complement regulatory proteins such as CFH (56). HO-1 may upregulate the expression of complement regulatory protein-decay acceleration factor and inhibit the production of complement C3 and C5 converting enzymes, minimizing the deposition of MAC and thus protecting endothelial cells from damage (57,58). N-acetylcysteine (NAC) is an agent, and recent studies suggest it may be a treatment for TTP. Li and colleagues provided the first clinical evidence for NAC’s efficacy in treating TTP (59). In addition, Cai et al. reported that NAC contributed to a substantial increase in HO-1 mRNA expression in carbon tetrachloride (CCl4)-injured livers (60). Our previous study showed that HO-1 levels were downregulated in TA-TMA patients, while in vitro experiments confirmed that HO-1 stimulants inhibited complement activation on endothelial cells. At the same time, NAC protected the endothelium from complement activation injury (61). Nuclear factor erythroid-associated factor 2 (Nrf-2) is one of the b-Zip transcription factors of the CNC (cap ‘n’ collar) family, which contains Nrf1, NF-E2, p45, and Nrf3 (62). In our recent investigation, Nrf2 levels were reduced in TA-TMA patients, and NAC was able to inhibit Nrf2/HO-1 levels, protect microvascular endothelial cells, and inhibit the development of TA-TMA (63). In addition, NAC can inhibit complement activation and vWF multimer formation (64). In another of our studies, we showed that upregulation of HIF-1α contributes to complement activation in TA-TMA. Comprehensive analysis, including DNA array, a luciferase reporter assay, chromatin immunoprecipitation (ChIP)-seq, and quantitative polymerase chain reaction (PCR), revealed that HIF-1α interacted with the promoter of complement factor H (CFH) to inhibit its transcription (65). Professor Sabulski et al. wrote a commentary stating that the above study provides a potential new mechanism for complement activation in the TA-TMA state and suggests a potentially viable animal model for TA-TMA. However, whether complement activation due to hypoxic injury is a cause or a consequence of TA-TMA still needs to be further confirmed by numerous studies (66).
Inflammation and TA-TMA
Neutrophil extracellular traps (NETs) may be a critical component between complement activation, endothelial injury, and TA-TMA pathogenesis. The concept of NETs was first introduced by the German scholar Volker Brinkmann in 2004 (67). Activated neutrophils generated an innate immune response to round up and kill invading pathogens (67). In addition, several other factors, including activated platelets, inflammatory stimuli, or compounds, can also induce the formation of NETs after neutrophil activation. Multiple factors during HSCT, such as pretreatment drugs, systemic irradiation, infection, GVHD, and calmodulin inhibitors, can cause endothelial cell injury. Neutrophils and activated platelets are recruited to the injury site after endothelial damage, and neutrophils and platelets form aggregates that induce NET formation (68).
In turn, neutrophil activation promotes the release of complement factors, which causes the formation of terminal complement MAC (69), forming an amplification endothelial injury loop. In severe cases of coronavirus disease 2019 (COVID-19), neutrophil activation was found in patients with COVID-19 combined with micro thrombosis. In the context of thrombosis, NETs can trap erythrocytes and activate platelets. Neutrophil elastase associated with NETs enhances tissue factor activity and thrombin production. Moreover, NETs can act as a scaffold for thrombosis and a coagulation activator through multiple mechanisms (70). Arai et al. first proposed that elevated levels of NETs are associated with the development of transplantation-related thrombotic microangiopathy and identified micro thrombosis and NETs formation in renal autopsies of TA-TMA patients (71). A clinical trial of 103 pediatric hematopoietic stem cell transplant patients demonstrated that in patients who subsequently developed TA-TMA, NETs levels were significantly elevated on day 14 post-transplantation. In addition, damaged endothelial cells released IL-8, inducing the release of NETs from neutrophils, which activated complement and microthrombus formation (72). NETs-mediated neutrophil involvement in endothelial injury and TA-TMA pathogenesis remains further investigated.
Diagnosis
The more accepted diagnosis of TMA is made using the published diagnostic criteria proposed by Cho et al. (73), which include: (I) elevated lactate dehydrogenase (LDH) above the upper limit of normal for age; (II) new onset thrombocytopenia, with a platelet count <50×109/L or a 50% reduction in platelet count; (III) new onset anemia, with hemoglobin below the lower limit of normal or anemia requiring transfusion support; (IV) microangiopathy, defined as the presence of lytic cells in peripheral blood or histological evidence of microangiopathy on tissue specimens, and (V) absence of coagulopathy and negative Coombs test. All laboratory criteria must be present simultaneously, and criteria 1–4 must be documented on at least two consecutive tests to be classified as positive. ADAMTS13 activity was measured in subjects with TMA to rule out the diagnosis of TTP. the date of diagnosis of TMA was defined as the first date on which all diagnostic criteria were met. In recent years, the diagnostic role of complement for TA-TMA has been reported, and Jodele et al. used complement sC5b-9 as one of the diagnostic indicators for TA-TMA (6).
Therapies
Overview
Since 2010, the treatment of TA-TMA has advanced due to a better understanding of the pathogenesis of TA-TMA as well as risk factors. Preventive measures during transplantation, such as optimizing pre-transplant pretreatment protocols, avoiding infection, and avoiding GVHD, can minimize endothelial injury. Aggressive transplant management is central to TA-TMA treatment, including preventing and reducing hypertension, minimizing blood transfusions, and prophylactic anti-infective therapy. Other approaches include TPE, discontinuation of calcineurin inhibitors and mammalian target of rapamycin inhibitors (CNI/mTOR), defibrillation, rituximab, and antioxidant treatment (NAC), and the complement inhibitor eculizumab. Clinical trials have explored new therapeutic approaches, including second-generation C5 monoclonal antibodies and mannitol-binding lectin-associated serine protease-2 inhibition (MASP-2). The therapeutic targets involved in TA-TMA-related mechanisms are shown in Figure 1. In conclusion, early identification of TA-TMA and early intervention to eliminate risk factors can lead to a better prognosis for patients (13,74).
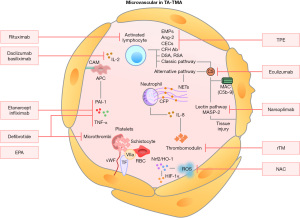
Withdrawal of immunosuppressive drugs
Previous studies have shown that CNI induces endothelial cell injury and promotes TA-TMA, so CNI is usually discontinued when TA-TMA is detected (19). Some studies even reported using specific drugs to clear CNI (8). However, the effectiveness of these measures is controversial (75). There is no evidence that TA-TMA onset, as well as severity, correlates with the concentration of immunosuppressive agents, such as cyclosporine and tacrolimus (76,77). Considering that some patients are notified of the presence of TA-TMA and GVHD, withdrawal of CNI should be made with caution, as it may exacerbate GVHD, and switching to other anti-GVHD drugs may be a practical option. Daclizumab, an IL-2 inhibitor, was shown by Wolff et al. to replace other anti-GVHD drugs while promoting TA-TMA remission. The study included 13 patients; nine had remission of TA-TMA, two were stable, and one had no response. However, the GVHD-related mortality was high in the entire cohort; therefore, this approach is currently pending additional evidence to confirm (78).
TPE
TA-TMA has limited efficacy for treatment by TPE because it is fundamentally different from TTP, with no significant differences in ADAMTS13 levels, and not all patients have detectable antibodies to the Complement H factor. A prospective cohort study involving TPE for TA-TMA showed an overall response rate of 64% for TPE (79). In another single-center retrospective study involving 66 patients with TA-TMA, 60% of the 63 patients treated with TPE had a response. And the 6-month cumulative survival was 50% for patients who responded and 0% for those who did not (77). A study of 15 patients with TA-TMA displayed that TPE did not prevent TA-TMA-related renal insufficiency (78).
Due to its clearance properties, TPE may be effective when complement H factor antibodies are present in patients (74). Early TA-TMA diagnosis and timely TPE application may result in a better outcome. A study of TA-TMA in children showed that earlier initiation of TPE resulted in a higher response rate (74). TPE-related complications such as infection, bleeding, and electrolyte disturbances may lead to worse outcomes with TPE, as suggested by some studies (19,80). Therefore, once TA-TMA is diagnosed, do not use TPE blindly, but only in the absence of other complement-blocking therapies or in the clear presence of complement H factor antibodies and when treatment can be initiated early during TA-TMA. According to the AFP guidelines, TPE is a weak grade 2C recommendation due to the poor quality of the evidence (81).
Rituximab
Rituximab, an anti-CD20 antibody, depletes the body of abnormal antibodies and performs immunomodulation (82). In combination with TPE, rituximab is highly effective in treating TTP (83). However, the evidence for TA-TMA was limited to a few cases, reports (84-91), and only some patients showed a positive response to rituximab.
Defibrotide
Defibrillation has a protective effect on microvascular endothelial cells and the ability to restore the thrombus-fibrinolytic balance in small vessels; therefore, it is approved for the treatment of TA-TMA (92). An investigation in Spain included 17 adult TA-TMA patients, five of whom were treated with defibrillation monotherapy and the control group with other therapies. Compared to the control group, 65% of patients in the defibrillation group were wholly cured with an OS of 59% (93). Yeates et al. also included 17 patients with TA-TMA in another study, and 76% achieved remission after defibrillation (94). Another study observed a 61% response rate in patients treated with defibrillation in combination with TPE or melphalan (92).
Recombinant thrombomodulin (rTM)
Thrombomodulin is a transmembrane glycoprotein with vascular endothelial cell-protective, antifibrinolytic, antithrombotic, and anti-inflammatory properties (95). Activated neutrophils cleave thrombomodulin upon endothelial cell activation, thus compromising the original vascular protective function. RTM has shown inconsistent results in multiple clinical trials investigating the effects on disseminated intravascular coagulation and sepsis (96,97). In case reports or small sample size analyses, rTM has been used in treating TA-TMA with mixed responses (71,98). Although the biological characteristics of rTM make it an appropriate treatment, there are limited data to support its use in TA-TMA.
Complement blockage
Eculizumab is a monoclonal anti-C5 antibody that blocks the complement pathway and inhibits the formation of complement C5b-9. Most published studies on eculizumab for TA-TMA treatment are retrospective cohorts with small sample sizes. Many studies included patients previously treated with rituximab and TPE, some of whom withdrew CNI/mTOR. Therefore, there were many confounding factors and insufficient evidence for conclusions. In addition, the treatment initiation timing in these patients varied widely. While most studies followed the dosing criteria for eculizumab in aHUS, which was 900 mg per week for four weeks and then 1,200 mg every two weeks, some studies dosed eculizumab on its drug concentration and complement levels. Overall, these studies’ response rates in TA-TMA patients ranged from 50–93%, with overall OS rates ranging from 33–60% (12,14,15,99-102). One of these groups observed that eculizumab needs to be applied promptly at an early stage of TA-TMA onset for a better outcome (101). Jodele et al. recently published a study on using eculizumab in 64 pediatric patients under 18 years old with high-risk TA-TMA. The overall response rate was 64%, with a 1-year OS of 66%. Of the patients who died, only 2 achieved remission of TA-TMA, but the remaining 27 had signs of active TA-TMA at the time of death (13). It should be noted that eculizumab is administered dynamically based on eculizumab blood concentrations and CH50 levels.
We conducted a meta-analysis of the efficacy and safety of eculizumab in treating TA-TMA. We included six studies involving 116 patients. Meta-analysis revealed that the pooled estimates of complete response rate (CRR), overall response rate (ORR), and survival rate (SR) in TA-TMA patients were 32% (95% CI: 11–56%), 71% (95% CI: 58–82%) and 52% (95% CI: 40–65%), respectively. Infection was the most common adverse effect (103). Eculizumab improved ORR and SR in TA-TMA patients with few adverse events. However, limited by sample size and retrospective studies, the current findings remain highly biased. Therefore, higher-quality randomized controlled trials are needed to validate the efficacy and prognosis.
However, eculizumab has not been approved by the FDA for TA-TMA. Although the FDA currently supports complement-directed therapy for microangiopathic hematocrit anemia, particularly paroxysmal nocturnal hemoglobinuria and aHUS, its efficacy in TA-TMA has been demonstrated in several studies. The use of complement blockers is logical due to the role of complement in TA-TMA. Despite encouraging preliminary findings, a survey of the anti-C5 compound LFG316 was stopped early after results in seven patients showed a low likelihood of clinical benefit (NCT02763644).
MASP-2 inhibition
The MASP-2 inhibitor narsoplimab (OMS721) is a novel selective complement inhibitor that effectively blocks abnormal complement activation and has a protective effect on endothelial cells with minimal impact on immune function. Its ability to act on mannose-binding lectin-associated serine protease-2 affects the process of complement activation. Since it blocks only one of the three complement pathways, this contributes to its ability to preserve the respective functions of the other innate immunity pathways and can avoid widespread damage (16). A clinical trial initiated by Khaled et al. (NCT02222545) included 28 patients with TA-TMA and showed that the overall response rate after applying narsoplimab was 61%. Similar responses were observed in all patient subgroups defined according to baseline characteristics, HSCT characteristics, and complications. Seventy-four percent of patients had improved organ function. One-hundred-day SRs after HSCT-TMA diagnosis were 94% and 68% for responders, respectively, with a median OS of 274 days. Narsoplimab was well tolerated, and adverse events in this population were typical, with no significant safety concerns (104).
N-acetyl-L-cysteine
Alterations in the oxidative microenvironment play an essential role in the pathogenesis of TA-TMA. A study by Chen et al. illustrated that NAC reduced vWF multimers and inhibited platelet aggregation and collagen binding, suggesting that NAC may have therapeutic value in TA-TMA (105). Subsequently, Rottenstreich et al. reported a group of three patients with TTP who successfully achieved remission by adding NAC to standard therapy (106). We have previously also addressed the first complete report of a 36-year-old female TA-TMA patient successfully cured with NAC and explored the pathogenesis underpinning the efficacy (64). The results of our single-center clinical trial (NCT03252925) using NAC to prevent the occurrence of TA-TMA showed that the incidence of TA-TMA was 9.1% in the NAC cohort compared with 23% in the placebo cohort. The median time to TA-TMA onset was 60 and 36 days in the NAC and control groups, respectively. The 2-year OS rates were 75.4% and 63.0% in the NAC and placebo groups, respectively, with a hazard ratio (HR) of 0.622. the EFS rate was 25.8% in NAC patients and 8.1% in placebo patients, with an HR of 0.254. They suggested that NAC effectively prevents the occurrence of TA-TMA (107). However, there are fewer studies on NAC for TA-TMA, and more clinical trials are needed to validate it in the future.
Strengths and laminations
This review summarizes the pathophysiological processes as well as the diagnosis and treatment of TA-TMA and highlights the effects of inflammation and oxidative microenvironment on TA-TMA, which have not been summarized in previous reviews and which are part of several previous studies by the authors of this article. However, the question of whether the inflammatory and oxidative microenvironment is a cause or a consequence of the development of TA-TMA remains unexplained, and more studies are expected to elucidate this disease in the future.
Conclusion and prospects
TA-TMA is a severe complication after HSCT with high morbidity and mortality, for which the pathogenesis is not yet clear, and treatments are not fully effective. Many new therapies include new complement blockers and emerging prevention methods. Further elucidation of the disease’s risk factors and pathophysiology will provide the theoretical basis for additional prevention and treatment options. Advances in treatment also depend on rapid and accurate diagnosis, which requires a uniform standard. Many novel therapies will also need to be validated in multicenter, large sample size clinical trials.
Acknowledgments
Funding: This work was supported by the National Natural Science Foundation of China (81873432 and 82070143), grants from the Jiangsu Province of China (BE2021645), the National clinical research center for hematologic diseases (2021ZKQA01), and the Priority Academic Program Development of Jiangsu Higher Education Institutions (PAPD) (2018ZACQ02).
Footnote
Provenance and Peer Review: This article was commissioned by the Guest Editor (X. Long Zheng) for the series “Thrombotic Thrombocytopenic Purpura” published in Annals of Blood. The article has undergone external peer review.
Reporting Checklist: The authors have completed the Narrative Review reporting checklist (available at https://aob.amegroups.com/article/view/10.21037/aob-22-22/rc).
Conflicts of Interest: Both authors have completed the ICMJE uniform disclosure form (available at https://aob.amegroups.com/article/view/10.21037/aob-22-22/coif). The series “Thrombotic Thrombocytopenic Purpura” was commissioned by the editorial office without any funding or sponsorship. The authors have no other conflicts of interest to declare.
Ethical Statement: The authors are accountable for all aspects of the work in ensuring that questions related to the accuracy or integrity of any part of the work are appropriately investigated and resolved.
Open Access Statement: This is an Open Access article distributed in accordance with the Creative Commons Attribution-NonCommercial-NoDerivs 4.0 International License (CC BY-NC-ND 4.0), which permits the non-commercial replication and distribution of the article with the strict proviso that no changes or edits are made and the original work is properly cited (including links to both the formal publication through the relevant DOI and the license). See: https://creativecommons.org/licenses/by-nc-nd/4.0/.
References
- Jodele S, Medvedovic M, Luebbering N, et al. Interferon-complement loop in transplant-associated thrombotic microangiopathy. Blood Adv 2020;4:1166-77. [Crossref] [PubMed]
- Schoettler M, Lehmann LE, Margossian S, et al. Risk factors for transplant-associated thrombotic microangiopathy and mortality in a pediatric cohort. Blood Adv 2020;4:2536-47. [Crossref] [PubMed]
- Pagliuca S, Michonneau D, Sicre de Fontbrune F, et al. Allogeneic reactivity-mediated endothelial cell complications after HSCT: a plea for consensual definitions. Blood Adv 2019;3:2424-35. [Crossref] [PubMed]
- Laskin BL, Goebel J, Davies SM, et al. Small vessels, big trouble in the kidneys and beyond: hematopoietic stem cell transplantation-associated thrombotic microangiopathy. Blood 2011;118:1452-62. [Crossref] [PubMed]
- Dvorak CC, Higham C, Shimano KA. Transplant-Associated Thrombotic Microangiopathy in Pediatric Hematopoietic Cell Transplant Recipients: A Practical Approach to Diagnosis and Management. Front Pediatr 2019;7:133. [Crossref] [PubMed]
- Jodele S, Davies SM, Lane A, et al. Diagnostic and risk criteria for HSCT-associated thrombotic microangiopathy: a study in children and young adults. Blood 2014;124:645-53. [Crossref] [PubMed]
- Jodele S. Complement in Pathophysiology and Treatment of Transplant-Associated Thrombotic Microangiopathies. Semin Hematol 2018;55:159-66. [Crossref] [PubMed]
- García-Martín P, Alarcón-Payer C, López-Fernández E, et al. Transplantation-Associated Thrombotic Microangiopathy in Patients Treated With Sirolimus and Cyclosporine as Salvage Therapy for Graft-Versus-Host Disease. Ann Pharmacother 2015;49:986-94. [Crossref] [PubMed]
- Carreras E. Vascular endothelial syndromes after HCT: 2020 update. Bone Marrow Transplant 2020;55:1885-7. [Crossref] [PubMed]
- Postalcioglu M, Kim HT, Obut F, et al. Impact of Thrombotic Microangiopathy on Renal Outcomes and Survival after Hematopoietic Stem Cell Transplantation. Biol Blood Marrow Transplant 2018;24:2344-53. [Crossref] [PubMed]
- Gavriilaki E, Sakellari I, Batsis I, et al. Transplant-associated thrombotic microangiopathy: Incidence, prognostic factors, morbidity, and mortality in allogeneic hematopoietic cell transplantation. Clin Transplant 2018;32:e13371. [Crossref] [PubMed]
- Vasu S, Wu H, Satoskar A, et al. Eculizumab therapy in adults with allogeneic hematopoietic cell transplant-associated thrombotic microangiopathy. Bone Marrow Transplant 2016;51:1241-4. [Crossref] [PubMed]
- Jodele S, Dandoy CE, Lane A, et al. Complement blockade for TA-TMA: lessons learned from a large pediatric cohort treated with eculizumab. Blood 2020;135:1049-57. [PubMed]
- de Fontbrune FS, Galambrun C, Sirvent A, et al. Use of Eculizumab in Patients With Allogeneic Stem Cell Transplant-Associated Thrombotic Microangiopathy: A Study From the SFGM-TC. Transplantation 2015;99:1953-9. [Crossref] [PubMed]
- Rudoni J, Jan A, Hosing C, et al. Eculizumab for transplant-associated thrombotic microangiopathy in adult allogeneic stem cell transplant recipients. Eur J Haematol 2018;101:389-98. [Crossref] [PubMed]
- Patriarca F, Cigana C, Massimo D, et al. Risk Factors and Outcomes of Infections by Multidrug-Resistant Gram-Negative Bacteria in Patients Undergoing Hematopoietic Stem Cell Transplantation. Biol Blood Marrow Transplant 2017;23:333-9. [Crossref] [PubMed]
- Schoettler M, Chonat S, Williams K, et al. Emerging therapeutic and preventive approaches to transplant-associated thrombotic microangiopathy. Curr Opin Hematol 2021;28:408-16. [Crossref] [PubMed]
- Khosla J, Yeh AC, Spitzer TR, et al. Hematopoietic stem cell transplant-associated thrombotic microangiopathy: current paradigm and novel therapies. Bone Marrow Transplant 2018;53:129-37. [Crossref] [PubMed]
- Ho VT, Cutler C, Carter S, et al. Blood and marrow transplant clinical trials network toxicity committee consensus summary: thrombotic microangiopathy after hematopoietic stem cell transplantation. Biol Blood Marrow Transplant 2005;11:571-5. [Crossref] [PubMed]
- Peyvandi F, Siboni SM, Lambertenghi Deliliers D, et al. Prospective study on the behaviour of the metalloprotease ADAMTS13 and of von Willebrand factor after bone marrow transplantation. Br J Haematol 2006;134:187-95. [Crossref] [PubMed]
- Nürnberger W, Michelmann I, Burdach S, et al. Endothelial dysfunction after bone marrow transplantation: increase of soluble thrombomodulin and PAI-1 in patients with multiple transplant-related complications. Ann Hematol 1998;76:61-5. [Crossref] [PubMed]
- Cohen H, Bull HA, Seddon A, et al. Vascular endothelial cell function and ultrastructure in thrombotic microangiopathy following allogeneic bone marrow transplantation. Eur J Haematol 1989;43:207-14. [Crossref] [PubMed]
- Thachil J. Nitric oxide in transplantation-related thrombotic microangiopathy. Bone Marrow Transplant 2009;43:513-4. [Crossref] [PubMed]
- Goldberg RJ, Nakagawa T, Johnson RJ, et al. The role of endothelial cell injury in thrombotic microangiopathy. Am J Kidney Dis 2010;56:1168-74. [Crossref] [PubMed]
- Qi J, Wang J, Chen J, et al. Plasma levels of complement activation fragments C3b and sC5b-9 significantly increased in patients with thrombotic microangiopathy after allogeneic stem cell transplantation. Ann Hematol 2017;96:1849-55. [Crossref] [PubMed]
- Zhang Z, Wang H, Qi J, et al. Acute graft-versus-host disease increase risk and accuracy in prediction model of transplantation-associated thrombotic microangiopathy in patients with myelodysplastic syndrome. Ann Hematol 2022;101:1295-309. [Crossref] [PubMed]
- Scully M, Brown J, Patel R, et al. Human leukocyte antigen association in idiopathic thrombotic thrombocytopenic purpura: evidence for an immunogenetic link. J Thromb Haemost 2010;8:257-62. [Crossref] [PubMed]
- John ML, Hitzler W, Scharrer I. The role of human leukocyte antigens as predisposing and/or protective factors in patients with idiopathic thrombotic thrombocytopenic purpura. Ann Hematol 2012;91:507-10. [Crossref] [PubMed]
- Balassa K, Andrikovics H, Remenyi P, et al. The potential role of HLA-DRB1*11 in the development and outcome of haematopoietic stem cell transplantation-associated thrombotic microangiopathy. Bone Marrow Transplant 2015;50:1321-5. [Crossref] [PubMed]
- Jodele S, Laskin BL, Dandoy CE, et al. A new paradigm: Diagnosis and management of HSCT-associated thrombotic microangiopathy as multi-system endothelial injury. Blood Rev 2015;29:191-204. [Crossref] [PubMed]
- Jodele S, Licht C, Goebel J, et al. Abnormalities in the alternative pathway of complement in children with hematopoietic stem cell transplant-associated thrombotic microangiopathy. Blood 2013;122:2003-7. [Crossref] [PubMed]
- Holmes LV, Strain L, Staniforth SJ, et al. Determining the population frequency of the CFHR3/CFHR1 deletion at 1q32. PLoS One 2013;8:e60352. [Crossref] [PubMed]
- Tati R, Kristoffersson AC, Ståhl AL, et al. Complement activation associated with ADAMTS13 deficiency in human and murine thrombotic microangiopathy. J Immunol 2013;191:2184-93. [Crossref] [PubMed]
- Cataland SR, Holers VM, Geyer S, et al. Biomarkers of terminal complement activation confirm the diagnosis of aHUS and differentiate aHUS from TTP. Blood 2014;123:3733-8. [Crossref] [PubMed]
- Réti M, Farkas P, Csuka D, et al. Complement activation in thrombotic thrombocytopenic purpura. J Thromb Haemost 2012;10:791-8. [Crossref] [PubMed]
- Orth D, Khan AB, Naim A, et al. Shiga toxin activates complement and binds factor H: evidence for an active role of complement in hemolytic uremic syndrome. J Immunol 2009;182:6394-400. [Crossref] [PubMed]
- Thurman JM, Marians R, Emlen W, et al. Alternative pathway of complement in children with diarrhea-associated hemolytic uremic syndrome. Clin J Am Soc Nephrol 2009;4:1920-4. [Crossref] [PubMed]
- Lapeyraque AL, Malina M, Fremeaux-Bacchi V, et al. Eculizumab in severe Shiga-toxin-associated HUS. N Engl J Med 2011;364:2561-3. [Crossref] [PubMed]
- Menne J, Nitschke M, Stingele R, et al. Validation of treatment strategies for enterohaemorrhagic Escherichia coli O104:H4 induced haemolytic uraemic syndrome: case-control study. BMJ 2012;345:e4565. [Crossref] [PubMed]
- Noris M, Mescia F, Remuzzi G. STEC-HUS, atypical HUS and TTP are all diseases of complement activation. Nat Rev Nephrol 2012;8:622-33. [Crossref] [PubMed]
- Nadasdy T. Thrombotic microangiopathy in renal allografts: the diagnostic challenge. Curr Opin Organ Transplant 2014;19:283-92. [Crossref] [PubMed]
- Song D, Wu LH, Wang FM, et al. The spectrum of renal thrombotic microangiopathy in lupus nephritis. Arthritis Res Ther 2013;15:R12. [Crossref] [PubMed]
- Crovetto F, Borsa N, Acaia B, et al. The genetics of the alternative pathway of complement in the pathogenesis of HELLP syndrome. J Matern Fetal Neonatal Med 2012;25:2322-5. [Crossref] [PubMed]
- Totina A, Iorember F, El-Dahr SS, et al. Atypical hemolytic-uremic syndrome in a child presenting with malignant hypertension. Clin Pediatr (Phila) 2013;52:183-6. [Crossref] [PubMed]
- Wang Q, Zennadi R. Oxidative Stress and Thrombosis during Aging: The Roles of Oxidative Stress in RBCs in Venous Thrombosis. Int J Mol Sci 2020;21:4259. [Crossref] [PubMed]
- Chen S, Su Y, Wang J. ROS-mediated platelet generation: a microenvironment-dependent manner for megakaryocyte proliferation, differentiation, and maturation. Cell Death Dis 2013;4:e722. [Crossref] [PubMed]
- Ayer A, Zarjou A, Agarwal A, et al. Heme Oxygenases in Cardiovascular Health and Disease. Physiol Rev 2016;96:1449-508. [Crossref] [PubMed]
- Czibik G, Derumeaux G, Sawaki D, et al. Heme oxygenase-1: an emerging therapeutic target to curb cardiac pathology. Basic Res Cardiol 2014;109:450. [Crossref] [PubMed]
- Zhao Y, Zhang L, Qiao Y, et al. Heme oxygenase-1 prevents cardiac dysfunction in streptozotocin-diabetic mice by reducing inflammation, oxidative stress, apoptosis and enhancing autophagy. PLoS One 2013;8:e75927. [Crossref] [PubMed]
- Ma J, Lau CK, Obed A, et al. A cell penetrating heme oxygenase protein protects heart graft against ischemia/reperfusion injury. Gene Ther 2009;16:320-8. [Crossref] [PubMed]
- Hinkel R, Lange P, Petersen B, et al. Heme Oxygenase-1 Gene Therapy Provides Cardioprotection Via Control of Post-Ischemic Inflammation: An Experimental Study in a Pre-Clinical Pig Model. J Am Coll Cardiol 2015;66:154-65. [Crossref] [PubMed]
- Liu Y, Jing F, Yi W, et al. HO-1(hi) patrolling monocytes protect against vaso-occlusion in sickle cell disease. Blood 2018;131:1600-10. [Crossref] [PubMed]
- Han Q, Li G, Mak JC, et al. The role of heme oxygenase-1 in the protection of chronic intermittent hypoxia-induced lung injury in vivo. Zhonghua Jie He He Hu Xi Za Zhi 2015;38:516-9. [PubMed]
- Petersen B, Ramackers W, Lucas-Hahn A, et al. Transgenic expression of human heme oxygenase-1 in pigs confers resistance against xenograft rejection during ex vivo perfusion of porcine kidneys. Xenotransplantation 2011;18:355-68. [Crossref] [PubMed]
- Novo G, Cappello F, Rizzo M, et al. Hsp60 and heme oxygenase-1 (Hsp32) in acute myocardial infarction. Transl Res 2011;157:285-92. [Crossref] [PubMed]
- Tu CF, Tai HC, Wu CP, et al. The in vitro protection of human decay accelerating factor and hDAF/heme oxygenase-1 transgenes in porcine aortic endothelial cells against sera of Formosan macaques. Transplant Proc 2010;42:2138-41. [Crossref] [PubMed]
- Kinderlerer AR, Pombo Gregoire I, Hamdulay SS, et al. Heme oxygenase-1 expression enhances vascular endothelial resistance to complement-mediated injury through induction of decay-accelerating factor: a role for increased bilirubin and ferritin. Blood 2009;113:1598-607. [Crossref] [PubMed]
- Detsika MG, Duann P, Atsaves V, et al. Heme Oxygenase 1 Up-Regulates Glomerular Decay Accelerating Factor Expression and Minimizes Complement Deposition and Injury. Am J Pathol 2016;186:2833-45. [Crossref] [PubMed]
- Li GW, Rambally S, Kamboj J, et al. Treatment of refractory thrombotic thrombocytopenic purpura with N-acetylcysteine: a case report. Transfusion 2014;54:1221-4. [Crossref] [PubMed]
- Cai Z, Lou Q, Wang F, et al. N-acetylcysteine protects against liver injure induced by carbon tetrachloride via activation of the Nrf2/HO-1 pathway. Int J Clin Exp Pathol 2015;8:8655-62. [PubMed]
- Pan T, Qi J, You T, et al. Circulating Heme Oxygenase-1 and Complement Activation in Transplant-Associated Thrombotic Microangiopathy. Biol Blood Marrow Transplant 2019;25:1486-91. [Crossref] [PubMed]
- Motohashi H, O’Connor T, Katsuoka F, et al. Integration and diversity of the regulatory network composed of Maf and CNC families of transcription factors. Gene 2002;294:1-12. [Crossref] [PubMed]
- Zhang R, Qi J, Zhou M, et al. Upregulation of Nrf2 Attenuates Oxidative Stress-Induced, Complement Activation-Associated Endothelial Injury and Apoptosis in Transplant-Associated Thrombotic Microangiopathy. Transplant Cell Ther 2021;27:758.e1-8. [Crossref] [PubMed]
- Qi J, Hu S, He X, et al. N-Acetyl-L-Cysteine Potentially Inhibits Complement Activation in Transplantation-Associated Thrombotic Microangiopathy. Transplant Cell Ther 2022;28:216.e1-5. [Crossref] [PubMed]
- Qi J, Pan T, You T, et al. Upregulation of HIF-1α contributes to complement activation in transplantation-associated thrombotic microangiopathy. Br J Haematol 2022;199:603-15. [Crossref] [PubMed]
- Sabulski A, Jodele S. What complements complement in transplant-associated thrombotic microangiopathy? Br J Haematol 2022;199:477-9. [Crossref] [PubMed]
- Brinkmann V, Reichard U, Goosmann C, et al. Neutrophil extracellular traps kill bacteria. Science 2004;303:1532-5. [Crossref] [PubMed]
- Ghasemzadeh M, Hosseini E. Intravascular leukocyte migration through platelet thrombi: directing leukocytes to sites of vascular injury. Thromb Haemost 2015;113:1224-35. [Crossref] [PubMed]
- Yuen J, Pluthero FG, Douda DN, et al. NETosing Neutrophils Activate Complement Both on Their Own NETs and Bacteria via Alternative and Non-alternative Pathways. Front Immunol 2016;7:137. [Crossref] [PubMed]
- McFadyen JD, Stevens H, Peter K. The Emerging Threat of (Micro)Thrombosis in COVID-19 and Its Therapeutic Implications. Circ Res 2020;127:571-87. [Crossref] [PubMed]
- Arai Y, Yamashita K, Mizugishi K, et al. Serum neutrophil extracellular trap levels predict thrombotic microangiopathy after allogeneic stem cell transplantation. Biol Blood Marrow Transplant 2013;19:1683-9. [Crossref] [PubMed]
- Gloude NJ, Khandelwal P, Luebbering N, et al. Circulating dsDNA, endothelial injury, and complement activation in thrombotic microangiopathy and GVHD. Blood 2017;130:1259-66. [Crossref] [PubMed]
- Cho BS, Yahng SA, Lee SE, et al. Validation of recently proposed consensus criteria for thrombotic microangiopathy after allogeneic hematopoietic stem-cell transplantation. Transplantation 2010;90:918-26. [Crossref] [PubMed]
- Jodele S, Laskin BL, Goebel J, et al. Does early initiation of therapeutic plasma exchange improve outcome in pediatric stem cell transplant-associated thrombotic microangiopathy? Transfusion 2013;53:661-7. [Crossref] [PubMed]
- Uderzo C, Bonanomi S, Busca A, et al. Risk factors and severe outcome in thrombotic microangiopathy after allogeneic hematopoietic stem cell transplantation. Transplantation 2006;82:638-44. [Crossref] [PubMed]
- Changsirikulchai S, Myerson D, Guthrie KA, et al. Renal thrombotic microangiopathy after hematopoietic cell transplant: role of GVHD in pathogenesis. Clin J Am Soc Nephrol 2009;4:345-53. [Crossref] [PubMed]
- Oran B, Donato M, Aleman A, et al. Transplant-associated microangiopathy in patients receiving tacrolimus following allogeneic stem cell transplantation: risk factors and response to treatment. Biol Blood Marrow Transplant 2007;13:469-77. [Crossref] [PubMed]
- Wolff D, Wilhelm S, Hahn J, et al. Replacement of calcineurin inhibitors with daclizumab in patients with transplantation-associated microangiopathy or renal insufficiency associated with graft-versus-host disease. Bone Marrow Transplant 2006;38:445-51. [Crossref] [PubMed]
- Laskin BL, Goebel J, Davies SM, et al. Early clinical indicators of transplant-associated thrombotic microangiopathy in pediatric neuroblastoma patients undergoing auto-SCT. Bone Marrow Transplant 2011;46:682-9. [Crossref] [PubMed]
- Epperla N, Li A, Logan B, et al. Incidence, Risk Factors for and Outcomes of Transplant-Associated Thrombotic Microangiopathy. Br J Haematol 2020;189:1171-81. [Crossref] [PubMed]
- Padmanabhan A, Connelly-Smith L, Aqui N, et al. Guidelines on the Use of Therapeutic Apheresis in Clinical Practice - Evidence-Based Approach from the Writing Committee of the American Society for Apheresis: The Eighth Special Issue. J Clin Apher 2019;34:171-354. [Crossref] [PubMed]
- Ravani P, Bonanni A, Rossi R, et al. Anti-CD20 Antibodies for Idiopathic Nephrotic Syndrome in Children. Clin J Am Soc Nephrol 2016;11:710-20. [Crossref] [PubMed]
- George JN. TTP: the evolution of clinical practice. Blood 2021;137:719-20. [Crossref] [PubMed]
- Vasko R, Groenewold F, Korsten P, et al. Plasmapheresis-refractory thrombotic microangiopathy in a hematopoietic stem cell transplant recipient. Ther Apher Dial 2011;15:507-9. [Crossref] [PubMed]
- Carella AM, D’Arena G, Greco MM, et al. Rituximab for allo-SCT-associated thrombotic thrombocytopenic purpura. Bone Marrow Transplant 2008;41:1063-5. [Crossref] [PubMed]
- Au WY, Ma ES, Lee TL, et al. Successful treatment of thrombotic microangiopathy after haematopoietic stem cell transplantation with rituximab. Br J Haematol 2007;137:475-8. [Crossref] [PubMed]
- Ostronoff M, Ostronoff F, Calixto R, et al. Life-threatening hemolytic-uremic syndrome treated with rituximab in an allogeneic bone marrow transplant recipient. Bone Marrow Transplant 2007;39:649-51. [Crossref] [PubMed]
- Gallerani E, Lerch E, Romagnani E, et al. Thrombotic thrombocytopenic purpura associated with renal failure after autologous transplantation for multiple myeloma successfully treated with rituximab. Eur J Haematol 2006;77:527-9. [Crossref] [PubMed]
- Naina HV, Gertz MA, Elliott MA. Thrombotic microangiopathy during peripheral blood stem cell mobilization. J Clin Apher 2009;24:259-61. [Crossref] [PubMed]
- Simon S, Sandhu A, Selva D, et al. Bilateral trochlear nerve palsies following dorsal midbrain haemorrhage. N Z Med J 2009;122:72-5. [PubMed]
- Choi CM, Schmaier AH, Snell MR, et al. Thrombotic microangiopathy in haematopoietic stem cell transplantation: diagnosis and treatment. Drugs 2009;69:183-98. [Crossref] [PubMed]
- Kornblum N, Ayyanar K, Benimetskaya L, et al. Defibrotide, a polydisperse mixture of single-stranded phosphodiester oligonucleotides with lifesaving activity in severe hepatic veno-occlusive disease: clinical outcomes and potential mechanisms of action. Oligonucleotides 2006;16:105-14. [Crossref] [PubMed]
- Martínez-Muñoz ME, Forés R, Lario A, et al. Use of defibrotide to treat adult patients with transplant-associated thrombotic microangiopathy. Bone Marrow Transplant 2019;54:142-5. [Crossref] [PubMed]
- Yeates L, Slatter MA, Bonanomi S, et al. Use of defibrotide to treat transplant-associated thrombotic microangiopathy: a retrospective study of the Paediatric Diseases and Inborn Errors Working Parties of the European Society of Blood and Marrow Transplantation. Bone Marrow Transplant 2017;52:762-4. [Crossref] [PubMed]
- Grinsztejn B, Hoagland B, Moreira RI, et al. Retention, engagement, and adherence to pre-exposure prophylaxis for men who have sex with men and transgender women in PrEP Brasil: 48 week results of a demonstration study. Lancet HIV 2018;5:e136-45. [Crossref] [PubMed]
- Akbaraly TN, Singh-Manoux A, Dugravot A, et al. Association of Midlife Diet With Subsequent Risk for Dementia. JAMA 2019;321:957-68. [Crossref] [PubMed]
- Mori S, Ai T, Sera T, et al. Human Soluble Recombinant Thrombomodulin, ART-123, Resolved Early Phase Coagulopathies, but Did Not Significantly Alter the 28 Day Outcome in the Treatment of DIC Associated with Infectious Systemic Inflammatory Response Syndromes. J Clin Med 2019;8:1553. [Crossref] [PubMed]
- Fujiwara H, Maeda Y, Sando Y, et al. Treatment of thrombotic microangiopathy after hematopoietic stem cell transplantation with recombinant human soluble thrombomodulin. Transfusion 2016;56:886-92. [Crossref] [PubMed]
- Patriarca F, Cigana C, Dozzo M, et al. Erratum to: Risk Factors and Outcomes of Infections by Multidrug-Resistant Gram-Negative Bacteria in Patients Undergoing Hematopoietic Stem Cell Transplantation Biol Blood Marrow Transplant 2017;23:1040. [Biol Blood Marrow Transplant 2017;23:333-339]. [Crossref] [PubMed]
- Epperla N, Hemauer K, Hamadani M, et al. Impact of treatment and outcomes for patients with posttransplant drug-associated thrombotic microangiopathy. Transfusion 2017;57:2775-81. [Crossref] [PubMed]
- Jodele S, Fukuda T, Vinks A, et al. Eculizumab therapy in children with severe hematopoietic stem cell transplantation-associated thrombotic microangiopathy. Biol Blood Marrow Transplant 2014;20:518-25. [Crossref] [PubMed]
- Jodele S, Dandoy CE, Myers KC, et al. New approaches in the diagnosis, pathophysiology, and treatment of pediatric hematopoietic stem cell transplantation-associated thrombotic microangiopathy. Transfus Apher Sci 2016;54:181-90. [Crossref] [PubMed]
- Zhang R, Zhou M, Qi J, et al. Efficacy and Safety of Eculizumab in the Treatment of Transplant-Associated Thrombotic Microangiopathy: A Systematic Review and Meta-Analysis. Front Immunol 2020;11:564647. [Crossref] [PubMed]
- Khaled SK, Claes K, Goh YT, et al. Narsoplimab, a Mannan-Binding Lectin-Associated Serine Protease-2 Inhibitor, for the Treatment of Adult Hematopoietic Stem-Cell Transplantation-Associated Thrombotic Microangiopathy. J Clin Oncol 2022;40:2447-57. [Crossref] [PubMed]
- Chen J, Reheman A, Gushiken FC, et al. N-acetylcysteine reduces the size and activity of von Willebrand factor in human plasma and mice. J Clin Invest 2011;121:593-603. [Crossref] [PubMed]
- Rottenstreich A, Hochberg-Klein S, Rund D, et al. The role of N-acetylcysteine in the treatment of thrombotic thrombocytopenic purpura. J Thromb Thrombolysis 2016;41:678-83. [Crossref] [PubMed]
- Pan T, Qi J, Tang Y, et al. N-Acetylcysteine as Prophylactic Therapy for Transplantation-Associated Thrombotic Microangiopathy: A Randomized, Placebo-Controlled Trial. Transplant Cell Ther 2022;28:764.e1-e7. [Crossref] [PubMed]
Cite this article as: Qi J, Han Y. A narrative review of transplant-associated thrombotic microangiopathy: pathogenesis and novel therapies. Ann Blood 2023;8:25.