Animal models for thrombotic thrombocytopenic purpura: a narrative review
Introduction
Thrombotic thrombocytopenic purpura (TTP) is caused by a severe deficiency of plasma metalloprotease ADAMTS13 (A Disintegrin And Metalloprotease with ThromboSpondin type 1 repeats, 13) (1-5). ADAMTS13 is primarily synthesized in hepatic stellate cells (6-8) and endothelial cells (8-10), it is then released into the blood stream where it cleaves endothelium- and platelet-derived ultra-large (UL) von Willebrand factor (VWF) (11-13). The proteolysis of ULVWF is crucial for normal hemostasis and inhibition of inflammation (13-16). When the ability to cleave ULVWF is compromised, ULVWF multimers accumulate on endothelial surfaces or at the site of vascular injury where they recruit platelets from circulation, thus promoting the formation of occlusive thrombi in small arterioles and capillaries. This leads to systemic tissue ischemia and damage, the pathognomonic feature of TTP (11,17).
TTP is a rare but potentially fatal blood disorder, characterized by severe thrombocytopenia and microangiopathic hemolytic anemia with various degrees of organ dysfunction (18,19). Most TTP cases are caused by immunoglobulin G autoantibodies that bind and inhibit plasma ADAMTS13 (i.e., immune TTP or iTTP) (20-23). Rarely, TTP may also be caused by hereditary deficiency of plasma ADAMTS13 activity resulting from ADAMTS13 mutations (i.e., hereditary TTP or hTTP) (5,24-27). The mortality of TTP was ~90% when left unrecognized or untreated (18,28). Significant progress has been made in the past decades in terms of early diagnosis and therapeutic intervention for TTP, which has dramatically reduced the mortality and morbidity associated with the disease.
Therapeutic plasma exchange (TPE), in conjunction with corticosteroids, caplacizumab, rituximab, or other immunosuppressives, known as “triple therapy”, have been recommended by the International Society of Thrombosis and Haemostasis (ISTH) for all patients with new or relapsed iTTP (29). This combination of therapy has become the standard of care for iTTP (29-34). However, the mechanism underlying the onset, progression, exacerbation and/or relapse of TTP remains poorly understood.
This review will describe several animal models of TTP developed in past decades, which may provide tools for further assessing the potential environmental and/or genetic triggers of TTP and test potential novel therapeutics for TTP. We present this article in accordance with the Narrative Review reporting checklist (available at https://aob.amegroups.com/article/view/10.21037/aob-22-18/rc).
Methods
We performed a literature search through PubMed from 1969 to 2022 using free text: TTP and animal model (Table 1). We found a total of 67 peer-reviewed articles. Of these, 34 articles that did not discuss TTP models were excluded, and this gave us 33 articles for analysis and review. Additional articles were included based on historic reading database.
Table 1
Items | Specification |
---|---|
Date of search | September 25, 2022 |
Databases and other sources searched | PubMed |
Search terms used | Thrombotic thrombocytopenic purpura and animal model |
Timeframe | 1969–2022 |
Inclusion and exclusion criteria | Inclusion: peer-reviewed articles. Exclusion: no animal model used or discussed |
Selection process | Both authors conducted the selection with independent search |
Results
Hereditary TTP models
In hTTP, mutations in ADAMTS13 result in severe deficiency of plasma ADAMTS13 activity (2,5,24,27,35-38), primarily resulting from defective secretion of the ADAMTS13 protein (39). To model this, Adamts13 in animals was deleted to render ADAMTS13 nonfunctional. Both Adamts13-null mice and zebrafish models were generated with a classic homologous recombination through embryonic stem (ES) cells and CRISPR/cas gene editing approach, respectively. These animals were fully characterized and recapitulated some of the key clinical features of TTP in humans including thrombocytopenia, microangiopathic hemolytic anemia, and microvascular thrombosis in major organ tissue, etc. (Figure 1).
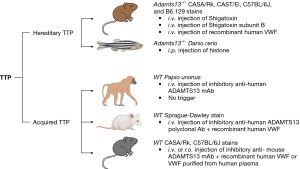
Mouse models
The first mouse model of TTP was established by Motto et al. (40) by deletion of several critical exons in the Adamts13 gene. Surprisingly, mice with nonfunctional Adamts13 (i.e., Adamts13-/-) in C57BL/6J background did not develop signs and symptoms consistent with TTP. However, after being crossed with CASA/Rk mice that have elevated plasma levels of VWF for several generations, the resulting new Adamts13-/- CASA/Rk mice developed spontaneous thrombocytopenia and showed a significantly decreased survival rate. Moreover, challenging these mice with a bacterial toxin, namely shigatoxin-2, could result in more profound and persistent thrombocytopenia, microangiopathic hemolytic anemia, and widespread VWF-rich thrombi in the small vessels, as well as an increased mortality rate (40). These are the classic features of TTP. These results recapitulate some of the features in human hTTP and suggest that severe ADAMTS13 deficiency alone may not be sufficient for the development of an acute episode of TTP; an additional environmental trigger or other genetic factors may contribute to the onset and progression of TTP resulting from severe ADAMTS13 deficiency.
This mouse model has been widely used by other investigators for characterizing the nature and mechanism of a potential environmental trigger (41), for studying the biological functions of ADAMTS13 for anti-thrombosis and anti-inflammation (12-16,42,43), and for testing potential novel therapeutics (44-47). For instance, Huang et al. (41) utilized the murine model to test which subunit of Shiga toxin is required for triggering TTP and what is the molecular mechanism underlying such a triggering effect. There are two variants of bacterial Shiga toxin: Stx1 and Stx2, each is composed of one catalytically active A subunit and 5 identical B subunits (48). While the A subunit is responsible for cytotoxicity of the toxin, B subunits mediate binding to cell-surface receptors (49). When injected into Adamts13-/- mice (CAST/Ei mice), the B subunits from either Stx1 or Stx2 appeared to be sufficient to trigger TTP (41), resulting from acute release of endothelial VWF (50,51).
To confirm whether an elevated plasma level of VWF is sufficient to trigger TTP when plasma ADAMTS13 is severely deficient, Schiviz et al. (52) reported the creation of a similar murine model. Again, no spontaneous TTP was observed in the Adamts13-/- mice. However, a single intravenous bolus of recombinant human VWF (rhVWF) (2,000 units/kg body weight) into Adamts13-/- mice rapidly induced a profound but transient thrombocytopenia. The platelet count returned to normal within one day of the rhVWF challenge. Despite the transient nature of thrombocytopenia, the presence of fragmentation of red blood cells (or schistocytosis), low hematocrit, and elevated serum lactate dehydrogenase (LDH) levels suggest the development of TTP in these mice following the rhVWF challenge. They further tested the efficacy of recombinant human ADAMTS13 (rhADAMTS13) in preventing or treating TTP in this model. As shown, an administration of rhADAMTS13 prior to or together with the rhVWF challenge protected Adamts13-/-mice from developing an acute TTP or at least reduced the severity of the disease.
To test the efficacy of adeno-associated virus serotype 8 (AAV8)-mediated gene therapy for hTTP, the Adamts13-/- mice generated by Dr. Motto (40) were utilized. Jin et al. (45) reported that administration of AAV8-hAAT-mdtcs (a truncated form of ADAMTS13) at doses greater than 2.6×1011 vg/kg body weight resulted in sustained expression of plasma ADAMTS13 activity at therapeutic levels. Expression of this truncated ADAMTS13 variant essentially eliminated the circulating ULVWF multimers, prevented severe thrombocytopenia, and reduced mortality in Adamts13-/- mice following a challenge with shigatoxin-2 (45). The data demonstrates the efficacy and supports the possibility of using the AAV-mediated gene therapy strategy for treatment of hereditary TTP in humans.
Zebrafish models
Zebrafish have gained their popularity and recognition as an excellent vertebrate animal model to study human blood diseases (53-55). The hemostasis-related genes including vwf and adamts13 in zebrafish are highly conserved with their corresponding orthologs in humans (56-58). Zebrafish thrombocytes, which are functionally equivalent to platelets, contain receptors that respond to human platelet agonists including thrombin, adenosinediphosphate (ADP), and collagen, etc. (59-61).
We reported the first TTP model in zebrafish created by CRISPR/Cas9 (62). The results demonstrated that adamts13-/- (null) zebrafish were viable, but with a 30% reduction in their total thrombocyte counts (62). An intraperitoneal administration of lysine-rich histone, a molecule of damage-associated molecular patterns (DAMPs) (63-65), into these zebrafish resulted in severe and persistent thrombocytopenia, fragmentation of erythrocytes, formation of VWF-rich microvascular thrombi, and increased mortality, consistent with the TTP phenotype (62). Moreover, a deletion of vwf (vwf-/-) essentially rescued either spontaneous thrombocytopenia or histone-induced TTP in adamts13-/- zebrafish (62), further confirming the critical roles of ADAMTS13 and VWF in the pathogenesis of TTP (Figure 1). These zebrafish models will be used to discover the role and mechanism of novel environmental factors or genetic modifiers in the pathogenesis of TTP.
Acquired TTP models
To date, there is no animal of true autoimmune TTP beside humans. In humans, TTP is primarily caused by IgG type autoantibodies against ADAMTS13 (21-23,66-73). Risk factors for the development of such autoantibodies are not clear, although TTP is more commonly seen in young females, particularly of African descent (74,75).
To generate acquired deficiency of ADAMTS13, an inhibitory antibody (monoclonal or polyclonal) against ADAMTS13 was injected into a wild-type animal to inhibit plasma ADAMTS13 activity (Figure 1). Following antibody administration, plasma ADAMTS13 activity and the clinical features of TTP were assessed. Similar to the hTTP model, a certain environmental trigger was used to incite an acute episode of TTP, if animals with acquired deficiency of plasma ADAMTS13 did not develop the signs and laboratory evidence of TTP.
Baboon models
Feys et al. (76) reported the first non-human primate TTP model by repeated injections of an inhibitory monoclonal antibody (mAb) to the wild-type baboon (Papio ursinus). They found that a 4-day functional inhibition of plasma ADAMTS13 activity was sufficient to induce spontaneous TTP in baboons without an additional trigger. The baboons receiving inhibitory mAb presented with a characteristic hematologic picture of TTP, including severe thrombocytopenia, schistocytic hemolytic anemia, and a rapid rise in serum LDH activity; additionally, immunohistochemical studies revealed the presence of disseminated platelet- and VWF-rich thrombi in several major organs including kidneys, heart, brain, and spleen. Interestingly, these baboons did not develop a fatal condition (Figure 1). Nevertheless, these results indicate that there may be an unidentified genetic or environmental factor that confers baboons’ susceptibility to TTP when their plasma ADAMTS13 activity is profoundly inhibited. Additionally, the no-fatality resulting from the baboon model of TTP is consistent with the transient nature of supplemented inhibitory antibodies and some potential mechanisms present in baboons that confer a survival benefit in the case of acute TTP.
Rat models
Tersteeg et al. (77) reported the first rat model of TTP. A polyclonal antibody against ADAMTS13 (650 U/kg) was used to block plasma ADAMTS13 activity in wild-type Sprague-Dawley rats. When challenged with rhVWF (2,000 U/kg), these animals with antibody-mediated inhibition of plasma ADAMTS13 activity displayed severe “TTP-like” signs and symptoms, including thrombocytopenia, hemolytic anemia, and VWF-rich thrombi in the kidneys and brain. Again, a subsequent infusion of rhADAMTS13 (400, 800, or 1,600 U/kg) prevented the full development of “TTP-like” symptoms. The amount of rhADAMTS13 was able to override the injected amount of anti-ADAMTS13 antibodies, thus restoring plasma ADAMTS13 activity and allowing the normal degradation of ULVWF multimers to occur. The rat model may have different uses for investigating the biology of ADAMTS13 and its disease association in vivo.
Mouse models
In addition to the genetic mouse models, Deforche et al. (78) reported a mouse model with acquired ADAMTS13 deficiency. Through extensive screening of their monoclonal antibodies (mAbs) against murine ADAMTS13, they found four mAbs that strongly inhibited murine ADAMTS13 activity in vitro (~68–90% inhibition) using a fluorescent resonance energy transfers (FRETS)-VWF73 based assay. Two inhibitory mAbs (13B4 and 14H7) were injected into wild type mice at 1.25 mg/kg, each resulted in nearly complete inhibition of plasma ADAMTS13 activity (96%±4% inhibition, day 1 post injection). This led to the accumulation of ULVWF in murine plasma. Following a single bolus intravenous injection of these two mAbs, inhibition of murine plasma ADAMTS13 lasted for more than 7 days.
Like genetic deficiency of ADAMTS13 in Adamts13-/- mice, the mice with acquired inhibition of plasma ADAMTS13 activity did not develop spontaneous TTP either. However, 24 hours following an intravenous infusion of rhVWF (500 U/kg), these mice developed marked thrombocytopenia and elevation of serum LDH, consistent with the clinical features of TTP (78) (Figure 1).
To create a persistent acquired deficiency of plasma ADAMTS13 activity, Ostertag et al. (79) used a hydrodynamic injection of naked DNA encoding human anti-ADAMTS13 scFv4-20 that targets at the spacer domain. Ten days following the hydrodynamic injection of scFv4-20, plasma ADAMTS13 activity was completely absent and ULVWF multimers appeared in plasma and thrombus formation after laser injury in cremaster blood vessel was significantly enhanced. However, no spontaneous thrombocytopenia and hemolysis occurred. When an intravenous injection of Shigatoxin-2 was given to the mice at 50 ng/kg weight, all mice with severe ADAMTS13 deficiency developed severe thrombocytopenia and died within 5 days. Histology analysis revealed the presence of disseminated microvascular thrombosis in several major organs including brain, heart, and kidneys in the deceased mice. These results demonstrate for the first time the ability of a cloned human monoclonal recombinant monovalent antibody fragment against ADAMTS13 to recapitulate the key pathologic features of untreated acquired TTP in vivo, validating the clinical significance of isolated monoclonal antibodies and providing a useful animal model for testing novel targeted therapeutic approaches for TTP.
Conclusions
In summary, the TTP animal models created from zebrafish to non-human primates have helped researchers make important progress in understanding the disease mechanism and developing new therapeutics. While hTTP and iTTP are two distinct forms, in terms of the underlying etiology of severe ADAMTS13 deficiency, most iTTP cases also exhibit low levels of ADAMTS13 antigen resulting from accelerated clearance of ADAMTS13/antibody immune complexes. In this regard, iTTP resembles hTTP (80). Thus, both genetically modified and antibody-mediated animal models may be used to test the role of potential environmental factors such as infection and pregnancy or potential genetic modifiers such as complement factor H mutation (81-83) and ANRKD family proteins (84) in the pathogenesis of TTP. Further utilization of these TTP animal models will allow us to test the efficacy and safety of various targeted therapeutic strategies in addition to the restoration of plasma ADAMTS13 activity.
Prospective
Gene editing using the CRISPR/Cas system has made creation of animal models easier and more rapid than ever. Not only can we create an ADAMTS13 knockout animal mutant, but also generate a point-mutation in ADAMTS13 and other associated genetic modifiers that are found in patients with hTTP. Having a true hTTP model, rather than the ADAMTS13 null model, in conjunction with mutations in other genetic modifiers, will significantly enhance our ability to understand the biology of ADAMTS13 and the pathogenesis of TTP. Finally, we are still in urgent need of a true autoimmune TTP animal model, which may help our understanding of the immunological aspect of the disease, such as the triggers of ADAMTS13 autoantibody production and targeted immune therapies.
Acknowledgments
The authors thank Antonia Bignotti, Department of Pathology and Laboratory Medicine, University of Kansas Medical Center, for proofreading of the manuscript.
Funding: This work was supported in part by grants from National Blood Foundation (NBF 22-09R) (to LZ) and National Institutes of Health (HL144552 and HL157975) (to XLZ).
Footnote
Provenance and Peer Review: This article was commissioned by the editorial office, Annals of Blood for the series “Thrombotic Thrombocytopenic Purpura”. The article has undergone external peer review.
Reporting Checklist: The authors have completed the Narrative Review reporting checklist. Available at https://aob.amegroups.com/article/view/10.21037/aob-22-18/rc
Peer Review File: Available at https://aob.amegroups.com/article/view/10.21037/aob-22-18/prf
Conflicts of Interest: Both authors have completed the ICMJE uniform disclosure form (available at https://aob.amegroups.com/article/view/10.21037/aob-22-18/coif). The series “Thrombotic Thrombocytopenic Purpura” was commissioned by the editorial office without any funding or sponsorship. XLZ is a consultant for Alexion, Sanofi, BioMedica Diagnostics, Argenx, and Takeda, as well as a co-founder of Clotsolution. XLZ served as the unpaid Guest Editor of the series and serves as an unpaid editorial board member of Annals of Blood from March 2022 to February 2024. The authors have no other conflicts of interest to declare.
Ethical Statement: The authors are accountable for all aspects of the work in ensuring that questions related to the accuracy or integrity of any part of the work are appropriately investigated and resolved.
Open Access Statement: This is an Open Access article distributed in accordance with the Creative Commons Attribution-NonCommercial-NoDerivs 4.0 International License (CC BY-NC-ND 4.0), which permits the non-commercial replication and distribution of the article with the strict proviso that no changes or edits are made and the original work is properly cited (including links to both the formal publication through the relevant DOI and the license). See: https://creativecommons.org/licenses/by-nc-nd/4.0/.
References
- Zheng X, Majerus EM, Sadler JE. ADAMTS13 and TTP. Curr Opin Hematol 2002;9:389-94. [Crossref] [PubMed]
- Zheng XL, Sadler JE. Pathogenesis of thrombotic microangiopathies. Annu Rev Pathol 2008;3:249-77. [Crossref] [PubMed]
- Moake JL. von Willebrand factor, ADAMTS-13, and thrombotic thrombocytopenic purpura. Semin Hematol 2004;41:4-14. [Crossref] [PubMed]
- Zheng X, Chung D, Takayama TK, et al. Structure of von Willebrand factor-cleaving protease (ADAMTS13), a metalloprotease involved in thrombotic thrombocytopenic purpura. J Biol Chem 2001;276:41059-63. [Crossref] [PubMed]
- Levy GG, Nichols WC, Lian EC, et al. Mutations in a member of the ADAMTS gene family cause thrombotic thrombocytopenic purpura. Nature 2001;413:488-94. [Crossref] [PubMed]
- Uemura M, Tatsumi K, Matsumoto M, et al. Localization of ADAMTS13 to the stellate cells of human liver. Blood 2005;106:922-4. [Crossref] [PubMed]
- Zhou W, Inada M, Lee TP, et al. ADAMTS13 is expressed in hepatic stellate cells. Lab Invest 2005;85:780-8. [Crossref] [PubMed]
- Cao WJ, Niiya M, Zheng XW, et al. Inflammatory cytokines inhibit ADAMTS13 synthesis in hepatic stellate cells and endothelial cells. J Thromb Haemost 2008;6:1233-5. [Crossref] [PubMed]
- Turner N, Nolasco L, Tao Z, et al. Human endothelial cells synthesize and release ADAMTS-13. J Thromb Haemost 2006;4:1396-404. [Crossref] [PubMed]
- Kling SJ, Judd CA, Warner KB, et al. Regulation of ADAMTS13 expression in proliferating human umbilical vein endothelial cells. Pathophysiol Haemost Thromb 2008;36:233-40. [Crossref] [PubMed]
- Dong JF, Moake JL, Nolasco L, et al. ADAMTS-13 rapidly cleaves newly secreted ultralarge von Willebrand factor multimers on the endothelial surface under flowing conditions. Blood 2002;100:4033-9. [Crossref] [PubMed]
- Chauhan AK, Motto DG, Lamb CB, et al. Systemic antithrombotic effects of ADAMTS13. J Exp Med 2006;203:767-76. [Crossref] [PubMed]
- Chauhan AK, Kisucka J, Brill A, et al. ADAMTS13: a new link between thrombosis and inflammation. J Exp Med 2008;205:2065-74. [Crossref] [PubMed]
- Gandhi C, Khan MM, Lentz SR, et al. ADAMTS13 reduces vascular inflammation and the development of early atherosclerosis in mice. Blood 2012;119:2385-91. [Crossref] [PubMed]
- Khan MM, Motto DG, Lentz SR, et al. ADAMTS13 reduces VWF-mediated acute inflammation following focal cerebral ischemia in mice. J Thromb Haemost 2012;10:1665-71. [Crossref] [PubMed]
- Jin SY, Tohyama J, Bauer RC, et al. Genetic ablation of Adamts13 gene dramatically accelerates the formation of early atherosclerosis in a murine model. Arterioscler Thromb Vasc Biol 2012;32:1817-23. [Crossref] [PubMed]
- Arya M, Anvari B, Romo GM, et al. Ultralarge multimers of von Willebrand factor form spontaneous high-strength bonds with the platelet glycoprotein Ib-IX complex: studies using optical tweezers. Blood 2002;99:3971-7. [Crossref] [PubMed]
- Rock GA, Shumak KH, Buskard NA, et al. Comparison of plasma exchange with plasma infusion in the treatment of thrombotic thrombocytopenic purpura. Canadian Apheresis Study Group. N Engl J Med 1991;325:393-7. [Crossref] [PubMed]
- Zheng XL, Kaufman RM, Goodnough LT, et al. Effect of plasma exchange on plasma ADAMTS13 metalloprotease activity, inhibitor level, and clinical outcome in patients with idiopathic and nonidiopathic thrombotic thrombocytopenic purpura. Blood 2004;103:4043-9. [Crossref] [PubMed]
- Tsai HM. High titers of inhibitors of von Willebrand factor-cleaving metalloproteinase in a fatal case of acute thrombotic thrombocytopenic purpura. Am J Hematol 2000;65:251-5. [Crossref] [PubMed]
- Zheng X, Pallera AM, Goodnough LT, et al. Remission of chronic thrombotic thrombocytopenic purpura after treatment with cyclophosphamide and rituximab. Ann Intern Med 2003;138:105-8. [Crossref] [PubMed]
- Shelat SG, Smith P, Ai J, et al. Inhibitory autoantibodies against ADAMTS-13 in patients with thrombotic thrombocytopenic purpura bind ADAMTS-13 protease and may accelerate its clearance in vivo. J Thromb Haemost 2006;4:1707-17. [Crossref] [PubMed]
- Zheng XL, Wu HM, Shang D, et al. Multiple domains of ADAMTS13 are targeted by autoantibodies against ADAMTS13 in patients with acquired idiopathic thrombotic thrombocytopenic purpura. Haematologica 2010;95:1555-62. [Crossref] [PubMed]
- Kokame K, Matsumoto M, Soejima K, et al. Mutations and common polymorphisms in ADAMTS13 gene responsible for von Willebrand factor-cleaving protease activity. Proc Natl Acad Sci U S A 2002;99:11902-7. [Crossref] [PubMed]
- Manea M, Karpman D. Molecular basis of ADAMTS13 dysfunction in thrombotic thrombocytopenic purpura. Pediatr Nephrol 2009;24:447-58. [Crossref] [PubMed]
- Fujimura Y, Matsumoto M. Registry of 919 patients with thrombotic microangiopathies across Japan: database of Nara Medical University during 1998-2008. Intern Med 2010;49:7-15. [Crossref] [PubMed]
- van Dorland HA, Taleghani MM, Sakai K, et al. The International Hereditary Thrombotic Thrombocytopenic Purpura Registry: key findings at enrollment until 2017. Haematologica 2019;104:2107-15. [Crossref] [PubMed]
- Rock G, Shumak K, Kelton J, et al. Thrombotic thrombocytopenic purpura: outcome in 24 patients with renal impairment treated with plasma exchange. Canadian Apheresis Study Group. Transfusion 1992;32:710-4. [Crossref] [PubMed]
- Zheng XL, Vesely SK, Cataland SR, et al. ISTH guidelines for treatment of thrombotic thrombocytopenic purpura. J Thromb Haemost 2020;18:2496-502. [Crossref] [PubMed]
- Zheng XL. The standard of care for immune thrombotic thrombocytopenic purpura today. J Thromb Haemost 2021;19:1864-71. [Crossref] [PubMed]
- Abdelghany MT, Baggett MV. Caplacizumab for Acquired Thrombotic Thrombocytopenic Purpura. N Engl J Med 2016;374:2497. [Crossref] [PubMed]
- Scully M, Cataland SR, Peyvandi F, et al. Caplacizumab Treatment for Acquired Thrombotic Thrombocytopenic Purpura. N Engl J Med 2019;380:335-46. [Crossref] [PubMed]
- Dutt T, Shaw RJ, Stubbs MJ, et al. Real-World Evidence of Caplacizumab Use in the Management of Acute TTP. Blood 2021;137:1731-40. [Crossref] [PubMed]
- Zheng L, Zheng XL. How should caplacizumab be used for treatment of immune thrombotic thrombocytopenic purpura? Ann Blood 2023;8:11. [Crossref] [PubMed]
- Hommais A, Rayes J, Houllier A, et al. Molecular characterization of four ADAMTS13 mutations responsible for congenital thrombotic thrombocytopenic purpura (Upshaw-Schulman syndrome). Thromb Haemost 2007;98:593-9. [Crossref] [PubMed]
- Fujimura Y, Matsumoto M, Isonishi A, et al. Natural history of Upshaw-Schulman syndrome based on ADAMTS13 gene analysis in Japan. J Thromb Haemost 2011;9:283-301. [Crossref] [PubMed]
- Kinoshita S, Yoshioka A, Park YD, et al. Upshaw-Schulman syndrome revisited: a concept of congenital thrombotic thrombocytopenic purpura. Int J Hematol 2001;74:101-8. [Crossref] [PubMed]
- Matsumoto M, Kokame K, Soejima K, et al. Molecular characterization of ADAMTS13 gene mutations in Japanese patients with Upshaw-Schulman syndrome. Blood 2004;103:1305-10. [Crossref] [PubMed]
- Meyer SC, Jin SY, Cao WJ, et al. Characterization of five homozygous ADAMTS13 mutations in hereditary thrombotic thrombocyteopenic purpura-towards a phenotype-genotype correlation? Blood 2008;112:274. [Crossref]
- Motto DG, Chauhan AK, Zhu G, et al. Shigatoxin triggers thrombotic thrombocytopenic purpura in genetically susceptible ADAMTS13-deficient mice. J Clin Invest 2005;115:2752-61. [Crossref] [PubMed]
- Huang J, Motto DG, Bundle DR, et al. Shiga toxin B subunits induce VWF secretion by human endothelial cells and thrombotic microangiopathy in ADAMTS13-deficient mice. Blood 2010;116:3653-9. [Crossref] [PubMed]
- Chauhan AK, Walsh MT, Zhu G, et al. The combined roles of ADAMTS13 and VWF in murine models of TTP, endotoxemia, and thrombosis. Blood 2008;111:3452-7. [Crossref] [PubMed]
- Gandhi C, Motto DG, Jensen M, et al. ADAMTS13 deficiency exacerbates VWF-dependent acute myocardial ischemia/reperfusion injury in mice. Blood 2012;120:5224-30. [Crossref] [PubMed]
- Banno F, Chauhan AK, Kokame K, et al. The distal carboxyl-terminal domains of ADAMTS13 are required for regulation of in vivo thrombus formation. Blood 2009;113:5323-9. [Crossref] [PubMed]
- Jin SY, Xiao J, Bao J, et al. AAV-mediated expression of an ADAMTS13 variant prevents shigatoxin-induced thrombotic thrombocytopenic purpura. Blood 2013;121:3825-9, S1-3.
- Laje P, Shang D, Cao W, et al. Correction of murine ADAMTS13 deficiency by hematopoietic progenitor cell-mediated gene therapy. Blood 2009;113:2172-80. [Crossref] [PubMed]
- Niiya M, Endo M, Shang D, et al. Correction of ADAMTS13 deficiency by in utero gene transfer of lentiviral vector encoding ADAMTS13 genes. Mol Ther 2009;17:34-41. [Crossref] [PubMed]
- Bergan J, Dyve Lingelem AB, Simm R, et al. Shiga toxins. Toxicon 2012;60:1085-107. [Crossref] [PubMed]
- Beddoe T, Paton AW, Le Nours J, et al. Structure, biological functions and applications of the AB5 toxins. Trends Biochem Sci 2010;35:411-8. [Crossref] [PubMed]
- Liu F, Huang J, Sadler JE. Shiga toxin (Stx)1B and Stx2B induce von Willebrand factor secretion from human umbilical vein endothelial cells through different signaling pathways. Blood 2011;118:3392-8. [Crossref] [PubMed]
- Huang J, Haberichter SL, Sadler JE. The B subunits of Shiga-like toxins induce regulated VWF secretion in a phospholipase D1-dependent manner. Blood 2012;120:1143-9. [Crossref] [PubMed]
- Schiviz A, Wuersch K, Piskernik C, et al. A new mouse model mimicking thrombotic thrombocytopenic purpura: correction of symptoms by recombinant human ADAMTS13. Blood 2012;119:6128-35. [Crossref] [PubMed]
- Zhu XY, Liu HC, Guo SY, et al. A Zebrafish Thrombosis Model for Assessing Antithrombotic Drugs. Zebrafish 2016;13:335-44. [Crossref] [PubMed]
- Peng X, Dong M, Ma L, et al. A point mutation of zebrafish c-cbl gene in the ring finger domain produces a phenotype mimicking human myeloproliferative disease. Leukemia 2015;29:2355-65. [Crossref] [PubMed]
- Carradice D, Lieschke GJ. Zebrafish in hematology: sushi or science? Blood 2008;111:3331-42. [Crossref] [PubMed]
- Jin SY, Skipwith CG, Zheng XL. Amino acid residues Arg(659), Arg(660), and Tyr(661) in the spacer domain of ADAMTS13 are critical for cleavage of von Willebrand factor. Blood 2010;115:2300-10. [Crossref] [PubMed]
- Carrillo M, Kim S, Rajpurohit SK, et al. Zebrafish von Willebrand factor. Blood Cells Mol Dis 2010;45:326-33. [Crossref] [PubMed]
- Ghosh A, Vo A, Twiss BK, et al. Characterization of zebrafish von Willebrand factor reveals conservation of domain structure, multimerization, and intracellular storage. Adv Hematol 2012;2012:214209. [Crossref] [PubMed]
- Jagadeeswaran P, Sheehan JP, Craig FE, et al. Identification and characterization of zebrafish thrombocytes. Br J Haematol 1999;107:731-8. [Crossref] [PubMed]
- Jagadeeswaran P. Zebrafish: a tool to study hemostasis and thrombosis. Curr Opin Hematol 2005;12:149-52. [Crossref] [PubMed]
- Kretz CA, Weyand AC, Shavit JA. Modeling Disorders of Blood Coagulation in the Zebrafish. Curr Pathobiol Rep 2015;3:155-61. [Crossref] [PubMed]
- Zheng L, Abdelgawwad MS, Zhang D, et al. Histone-induced thrombotic thrombocytopenic purpura in adamts13 -/- zebrafish depends on von Willebrand factor. Haematologica 2020;105:1107-19. [Crossref] [PubMed]
- Kutcher ME, Xu J, Vilardi RF, et al. Extracellular histone release in response to traumatic injury: implications for a compensatory role of activated protein C. J Trauma Acute Care Surg 2012;73:1389-94. [Crossref] [PubMed]
- Fuchs TA, Kremer Hovinga JA, Schatzberg D, et al. Circulating DNA and myeloperoxidase indicate disease activity in patients with thrombotic microangiopathies. Blood 2012;120:1157-64. [Crossref] [PubMed]
- Sui J, Lu R, Halkidis K, et al. Plasma levels of S100A8/A9, histone/DNA complexes, and cell-free DNA predict adverse outcomes of immune thrombotic thrombocytopenic purpura. J Thromb Haemost 2021;19:370-9. [Crossref] [PubMed]
- George JN, Nester CM. Syndromes of thrombotic microangiopathy. N Engl J Med 2014;371:654-66. [Crossref] [PubMed]
- Tsai HM, Lian EC. Antibodies to von Willebrand factor-cleaving protease in acute thrombotic thrombocytopenic purpura. N Engl J Med 1998;339:1585-94. [Crossref] [PubMed]
- Klaus C, Plaimauer B, Studt JD, et al. Epitope mapping of ADAMTS13 autoantibodies in acquired thrombotic thrombocytopenic purpura. Blood 2004;103:4514-9. [Crossref] [PubMed]
- Luken BM, Turenhout EA, Hulstein JJ, et al. The spacer domain of ADAMTS13 contains a major binding site for antibodies in patients with thrombotic thrombocytopenic purpura. Thromb Haemost 2005;93:267-74. [Crossref] [PubMed]
- Luken BM, Kaijen PH, Turenhout EA, et al. Multiple B-cell clones producing antibodies directed to the spacer and disintegrin/thrombospondin type-1 repeat 1 (TSP1) of ADAMTS13 in a patient with acquired thrombotic thrombocytopenic purpura. J Thromb Haemost 2006;4:2355-64. [Crossref] [PubMed]
- Ferrari S, Scheiflinger F, Rieger M, et al. Prognostic value of anti-ADAMTS 13 antibody features (Ig isotype, titer, and inhibitory effect) in a cohort of 35 adult French patients undergoing a first episode of thrombotic microangiopathy with undetectable ADAMTS 13 activity. Blood 2007;109:2815-22. [Crossref] [PubMed]
- Ferrari S, Mudde GC, Rieger M, et al. IgG subclass distribution of anti-ADAMTS13 antibodies in patients with acquired thrombotic thrombocytopenic purpura. J Thromb Haemost 2009;7:1703-10. [Crossref] [PubMed]
- Pos W, Crawley JT, Fijnheer R, et al. An autoantibody epitope comprising residues R660, Y661, and Y665 in the ADAMTS13 spacer domain identifies a binding site for the A2 domain of VWF. Blood 2010;115:1640-9. [Crossref] [PubMed]
- Reese JA, Muthurajah DS, Kremer Hovinga JA, et al. Children and adults with thrombotic thrombocytopenic purpura associated with severe, acquired Adamts13 deficiency: comparison of incidence, demographic and clinical features. Pediatr Blood Cancer 2013;60:1676-82. [Crossref] [PubMed]
- Staley EM, Cao W, Pham HP, et al. Clinical factors and biomarkers predict outcome in patients with immune-mediated thrombotic thrombocytopenic purpura. Haematologica 2019;104:166-75. [Crossref] [PubMed]
- Feys HB, Roodt J, Vandeputte N, et al. Thrombotic thrombocytopenic purpura directly linked with ADAMTS13 inhibition in the baboon (Papio ursinus). Blood 2010;116:2005-10. [Crossref] [PubMed]
- Tersteeg C, Schiviz A, De Meyer SF, et al. Potential for Recombinant ADAMTS13 as an Effective Therapy for Acquired Thrombotic Thrombocytopenic Purpura. Arterioscler Thromb Vasc Biol 2015;35:2336-42. [Crossref] [PubMed]
- Deforche L, Tersteeg C, Roose E, et al. Generation of Anti-Murine ADAMTS13 Antibodies and Their Application in a Mouse Model for Acquired Thrombotic Thrombocytopenic Purpura. PLoS One 2016;11:e0160388. [Crossref] [PubMed]
- Ostertag EM, Bdeir K, Kacir S, et al. ADAMTS13 autoantibodies cloned from patients with acquired thrombotic thrombocytopenic purpura: 2. Pathogenicity in an animal model. Transfusion 2016;56:1775-85. [Crossref] [PubMed]
- Peyvandi F, Lavoretano S, Palla R, et al. ADAMTS13 and anti-ADAMTS13 antibodies as markers for recurrence of acquired thrombotic thrombocytopenic purpura during remission. Haematologica 2008;93:232-9. [Crossref] [PubMed]
- Noris M, Bucchioni S, Galbusera M, et al. Complement factor H mutation in familial thrombotic thrombocytopenic purpura with ADAMTS13 deficiency and renal involvement. J Am Soc Nephrol 2005;16:1177-83. [Crossref] [PubMed]
- Noris M, Mescia F, Remuzzi G. STEC-HUS, atypical HUS and TTP are all diseases of complement activation. Nat Rev Nephrol 2012;8:622-33. [Crossref] [PubMed]
- Zheng L, Zhang D, Cao W, et al. Synergistic effects of ADAMTS13 deficiency and complement activation in pathogenesis of thrombotic microangiopathy. Blood 2019;134:1095-105. [Crossref] [PubMed]
- Basu MK, Massicano F, Yu L, et al. Exome Sequencing Identifies Abnormalities in Glycosylation and ANKRD36C in Patients with Immune-Mediated Thrombotic Thrombocytopenic Purpura. Thromb Haemost 2021;121:506-17. [Crossref] [PubMed]
Cite this article as: Zheng L, Zheng XL. Animal models for thrombotic thrombocytopenic purpura: a narrative review. Ann Blood 2023;8:23.