Congenital and acquired disorders of secondary hemostasis
Introduction
Congenital and acquired disorders of secondary hemostasis often result in serious bleeding requiring subspecialty care by a hematologist. With advancements in understanding of the molecular underpinnings and relationships within secondary hemostasis, caring for patients with defects in secondary hemostasis continue to evolve and become increasingly complex requiring multidisciplinary care and expert clinical laboratories. While not exhaustive, this review attempts to outline and provide insight into congenital and acquired disorders of secondary hemostasis.
Congenital
Hemophilia A
Hemophilia A is a hereditary deficiency of factor VIII (FVIII), a critical component of secondary hemostasis (Figures 1,2). Without adequate levels of FVIII, there is inefficient production of thrombin and impaired hemostasis. Hemophilia A is an X-linked recessive disorder and most commonly presents in males during childhood, but it can also affect female carriers or females with Turner syndrome or skewed X-chromosome inactivation. In 30% of patients, it is caused by de novo mutations in the gene encoding FVIII. Clinical presentation correlates with the activity of FVIII in the plasma: mild (6–40%), moderate (1–5%), and severe (<1%) deficiencies (1). Hemophilia A occurs more frequently than hemophilia B, at a rate of approximately one in every 5,000 live male births, with a relatively higher incidence of severe disease (2). Patients may be identified by a prolonged activated partial thromboplastin time (aPTT) that corrects in a mixing study and a low FVIII activity. Treatment is focused on restoring FVIII activity. Initially, cryoprecipitate was used but resulted in transmission of blood-borne pathogens including hepatitis C and human immunodeficiency virus (HIV). Treatment advances continued with the advent of purified plasma-derived and recombinant FVIII and modifications to extend the factor half-life, maximizing the time between infusions while minimizing infectious risks. Those individuals with moderate-severe disease should receive prophylactic FVIII to minimize joint damage from hemarthrosis as standard of care, but all individuals with hemophilia A experiencing a bleed will receive on demand FVIII (3,4). Dosing is often based on actual body weight, although some have advocated for using ideal body weight or plasma volume-based calculations, especially with the growing frequency of obesity among individuals with hemophilia (5). Unfortunately, when some individuals with hemophilia A are treated with FVIII products, they develop inhibitory antibodies rendering infused FVIII inactive. In 2018, the Food and Drug Administration (FDA) approved emicizumab, a novel, long-acting subcutaneous injection that recapitulates the in vivo function of FVIII by facilitating the interaction of factor IX (FIX) and factor X (FX), even in the presence of a FVIII inhibitor (Figures 1,2). Emicizumab has quickly become a widely used agent for prophylaxis in patients with and without inhibitors in high resource settings. Importantly, emicizumab has been associated with the development of thrombotic microangiopathy when used with activated prothrombin complex concentrates (aPCC), requiring a thoughtful approach to breakthrough bleeding in those on prophylactic emicizumab with inhibitors. While these are the current mainstays of treatment, there are many others under investigation, including gene therapy and RNA interference technologies.
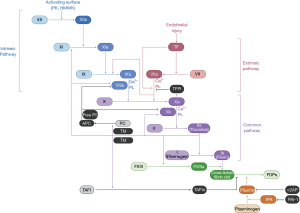
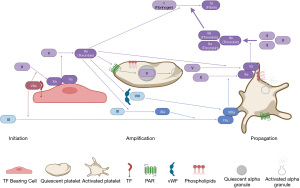
Hemophilia B
Hemophilia B is a hereditary deficiency of FIX that, like hemophilia A, leads to impaired thrombin generation and clinical bleeding (Figures 1,2). It is also X-linked recessive but is less prevalent, occurring in approximately one in every 25,000 live male births, with a relatively lower incidence of severe disease (2). Similar to hemophilia A, it can be diagnosed with the aPTT, mixing study, and a FIX activity level. Treatment focuses on restoring FIX activity, with the replacement of plasma-derived products or recombinant alternatives due to lower infection risk and modifications to extend half-life. Individuals with hemophilia B may also develop inhibitors when exposed to FIX products, although this occurs much less frequently than in hemophilia A and can be associated with anaphylactoid reactions. Future treatment options may also include gene therapies and RNA interference technologies.
Hemophilia C
Hemophilia C is a hereditary deficiency of factor XI (FXI) that is necessary for amplification of thrombin generation. Because initial thrombin generation can occur without FXI, patients have a milder and less predictable bleeding phenotype compared to hemophilia A and B (Figures 1,2). The F11 gene is located on chromosome 4 and inheritance is predominantly autosomal recessive, although it can rarely be autosomal dominant, with an increased prevalence in those of Ashkenazi Jewish ancestry (6). It is less common than hemophilia A and B, although cases are likely under-reported given the heterogeneity of presentation and possibility of mild bleeding. Deficiency of FXI is associated with bleeding particularly in anatomic locations with high fibrinolytic activity (e.g., oropharynx, genitourinary tract). Diagnosis is also made after a prolonged aPTT, mixing study, and FXI activity. Importantly, FXI activity does not directly correlate with clinical severity; thus, treatment is based on individuals’ bleeding history with prior hemostatic challenges. Since there is no FXI product in the United States, FXI repletion is accomplished with plasma transfusion, often in combination with anti-fibrinolytic agents (e.g., ε-aminocaproic acid, tranexamic acid).
Rare factor deficiencies
Factor II (FII) deficiency
Deficiency of FII, or prothrombin, is the least common congenital factor deficiency. Acquired causes of prothrombin deficiency include vitamin K deficiency, liver disease, and a rare antibody that enhances its clearance in individuals with antiphospholipid syndrome. Functioning within the “common pathway”, FII deficiency causes prolonged prothrombin time (PT) and aPTT, and it is confirmed with low FII activity or genetic evaluation. Treatment of bleeding relies on the use of PCC, and because of the long half-life of FII (3–4 days), requires only intermittent dosing (7).
Factor V (FV) deficiency
Deficiency of FV can be inherited, sometimes in conjunction with a FVIII deficiency, as well as acquired, especially from liver failure or, rarely, from a cross-reactive antibody that develops following exposure to older formulations of topical thrombin (7,8). FV deficiency results in prolonged PT and aPTT, as well as decreased FV activity. Treatment employs donor plasma or platelet concentrates, as large amounts of FV are stored within the platelet alpha granules; the half-life of FV is approximately 36 hours (Figure 2) (7).
Factor VII (FVII) deficiency
An isolated deficiency of FVII is typically inherited in an autosomal recessive fashion and acquired deficiency can be due to liver disease and vitamin K deficiency. Paradoxically, those with inherited FVII deficiency can develop thromboses, but often present with a spectrum of bleeding diatheses, including intracranial hemorrhage (9,10). Suspicion of FVII deficiency starts with a prolonged PT and a normal aPTT, as FVII is localized to the “extrinsic” pathway. The primary option to treat patients with FVII deficiency is recombinant FVIIa (rFVIIa) and antifibrinolytics, though other FVII-containing products (such as PCC) may be used if rFVIIa is not available; the half-life of FVII is approximately 4–6 hours (7).
FX deficiency
FX and FV form the prothrombinase complex that cleaves prothrombin to thrombin (Figures 1,2). Isolated deficiencies of FX are rare and may be inherited or acquired, classically in an individual with light chain amyloidosis where FX binds and is sequestered, but also in liver disease and vitamin K deficiency. Since FX is part of the “common pathway”, a deficiency results in a prolonged PT and aPTT. The FX activity in the plasma correlates well with clinical severity. There are FX concentrates available in the United States but if not readily accessible, then PCC or donor plasma may be used; the half-life of FX is approximately 40–60 hours (7).
Factor XIII (FXIII) deficiency
FXIII is a heterotetramer composed of two catalytic A subunits and two non-catalytic B subunits. It is necessary for in vivo coagulation, ensuring fibrin clot stability, but has little bearing on in vitro coagulation testing (Figure 1). Thus, individuals with FXIII deficiency have a normal PT and aPTT. Isolated FXIII deficiency may be inherited or acquired, classically presenting with recurrent miscarriages, poor wound healing, intracranial hemorrhage, and umbilical stump bleeding. Only if FXIII activity is extremely low or undetectable, the qualitative clot solubility test will be positive. Quantitative tests are also available and more sensitive. Genetic testing is used to confirm diagnosis and guide treatment based on the abnormality. The majority of mutations occur in the A subunit and can be treated with a recombinant concentrate containing only the A subunit, while pathogenic mutations in the B subunit are treated with a plasma-derived concentrate containing both subunits. If recombinant concentrates are unavailable, cryoprecipitate can be used. Since FXIII activity correlates with bleeding risk, those with very low levels often receive prophylaxis due to risk of spontaneous hemorrhage; given that the half-life of FXIII is 9–12 days, prophylactic dosing is administered infrequently (7,11,12).
Acquired
Oral drugs
Warfarin
Warfarin is a vitamin K antagonist that prevents hepatic gamma carboxylation of vitamin K-dependent coagulation factors FII, FVII, FIX, FX, protein C and protein S (13). Though warfarin is used less often in the direct oral anticoagulant (DOAC) era, its safety and efficacy are supported by robust clinical and research experience. Warfarin remains the standard of care for patients with mechanical heart valves, high-risk antiphospholipid antibody syndrome, and for patients for whom DOACs have been less efficacious. Warfarin is predominantly metabolized in the liver and has a half-life of 36–42 hours, though many variables influence its pharmacokinetics. Warfarin initiation classically results in a transient hypercoagulable state due to the relatively shorter half-life of protein C; for this reason, bridging therapy with another anticoagulant (i.e., heparin) may be indicated in patients with ongoing thrombosis or increased thrombotic risk. When chronic anticoagulation is interrupted for invasive procedures, bridging therapy may also be considered for patients with very high risk for thromboembolic events (14). Given warfarin’s effect on lowering FVII, the PT is increased soon after initiation of therapy, and its derived international normalized ratio (INR) is used to monitor dose adjustments. As with all anticoagulants, therapy may be complicated by bleeding ranging from minor to life-threatening. For patients who experience life-threatening bleeds while anticoagulated with warfarin, reversal can be quickly achieved and sustained using a combination of PCC and vitamin K (15,16).
Factor Xa (FXa) inhibitors
The oral FXa inhibitors (rivaroxaban, apixaban, edoxaban, betrixaban) are highly bioavailable inhibitors of circulating and clot bound FXa which impede formation of the prothrombinase complex. Their use is approved by the FDA for the prophylaxis and treatment of venous and arterial thrombosis. They each have unique pharmacokinetic properties, as outlined in their package inserts. They also generally have short half-lives, have some degree of renal clearance, and do not require routine monitoring. Chromogenic, heparin-calibrated anti-FXa assays may be employed to generally assess for drug presence or absence, but there are currently no established therapeutic parameters to allow for routine monitoring (17-19). For those patients who experience life-threatening bleeding, options for FXa inhibitor reversal include PCC or andexanet alfa (recombinant inactive FXa) in addition to supportive care (20-22). There is insufficient evidence to recommend one reversal strategy versus another, though availability and cost of andexanet alfa may limit its widespread use (16).
Direct thrombin inhibitors
Dabigatran is the only commercially available oral direct thrombin inhibitor and is similarly approved for treatment and prevention of venous and arterial thrombosis. It also has unique pharmacokinetic properties, as described in the package insert, and has a high degree of renal excretion which influences its use in different clinical scenarios. Dabigatran can prolong the PT only at maximal concentrations and only with sensitive reagents. Prolongation of aPTT is more reliable and linear with some reagents at therapeutic concentrations, such that the aPTT may be used in a semi-quantitative fashion for drug monitoring, though this is not routinely employed in practice (18). The thrombin time assay is highly sensitive to the presence of dabigatran and a normal result is sufficient to exclude its presence in the circulation. However, since even small concentrations of dabigatran can significantly prolong the thrombin time, the result does not correlate with drug concentration and anticoagulation efficacy (17-19). For patients who experience life-threatening bleeding requiring anticoagulation reversal, idarucizumab is recommended (16). Idarucizumab is a humanized monoclonal antibody fragment which acts rapidly to clear dabigatran from circulation. If idarucizumab is not available, alternate strategies include aPCC and PCC. Since factor concentrates carry prothrombotic risk, idarucizumab is highly favored, if available (16). Additional supportive care includes antifibrinolytic agents, activated charcoal if the last dose of dabigatran was within 2 hours, and potentially hemodialysis (16,21).
Parenteral drugs
Heparin
Unfractionated heparin is the backbone of anticoagulation in hospitalized patients. It is a sulfated glycosaminoglycan that complexes with the serine protease inhibitor antithrombin to enhance its ability to inactivate FXa and thrombin (23). Heparin is metabolized by the reticuloendothelial system and elimination at therapeutic doses is not substantially impacted by renal dysfunction. It causes prolongation of the aPTT in concentration-dependent fashion as well as appropriately calibrated chromogenic anti-Xa assays; the PT is not affected by heparin at clinical doses. However, aPTT may be confounded by other clinical factors (e.g., a lupus anticoagulant, liver dysfunction) (24). Because anti-Xa activity monitoring provides a more accurate evaluation of heparin anticoagulation, many institutions utilize titration protocols based on anti-Xa activity rather than the aPTT (25). After prolonged exposure, patients may develop antithrombin deficiency due to excessive consumption in addition to a general increase in heparin binding proteins during an acute phase reaction, which could explain “heparin resistance” (26,27). Heparin has the benefit of a rapid onset of action and a short half-life of approximately 1 hour. Protamine sulfate, a cation that sequesters highly anionic heparin molecules, is the agent of choice when rapid reversal is required (16).
Low molecular weight heparins (LMWH)
LMWHs (e.g., enoxaparin, dalteparin) consist of short chain polysaccharides as opposed to unfractionated heparin which contains chains of varying lengths. This difference results in preferential loss of thrombin inhibition yet continued FXa inhibition via enhanced antithrombin activity (28). LMWHs are injected subcutaneously, have a longer half-life, and have more predictable pharmacokinetics compared to unfractionated heparin. They are also predominantly cleared via the renal system, thus necessitating careful patient selection. In clinical scenarios where monitoring is warranted, a chromogenic anti-Xa activity assay is the test of choice (29). Reversal with protamine sulfate is incomplete due to inefficient binding of smaller polysaccharide chains and decreased ability to reverse FXa inhibition compared to thrombin inhibition (30,31).
Fondaparinux
Fondaparinux is a synthetic pentasaccharide which selectively binds with high affinity to antithrombin resulting in inhibition of FXa and the subsequent formation of the prothrombinase complex. It is approved for the prophylaxis and treatment of venous and arterial thrombosis and is an important therapeutic option for patients who cannot tolerate heparin products. Fondaparinux is administered subcutaneously and is predominantly renally cleared. There is no specific reversal agent for fondaparinux, although strategies that have been explored include rFVIIa, aPCC, and andexanet alfa. rFVIIa is most used based on ex vivo studies and case series but these are limited by lack of rigorous head-to-head comparison with other reversal strategies (15,32).
Direct thrombin inhibitors
Argatroban and bivalirudin are parenterally administered direct thrombin inhibitors which can bind to soluble and clot-bound thrombin. They are an important alternative to heparin in patients with suspected/proven heparin-induced thrombocytopenia. Both agents have a short half-life of under 1 hour. Argatroban is metabolized by the liver and bivalirudin by the kidneys. Like dabigatran, bivalirudin and argatroban prolong the aPTT in a concentration-dependent fashion. This effect is sufficiently rigorous to form the basis of protocols for dose titration (33). When bleeding complications occur, anticoagulant cessation is the primary intervention given the short half-life of these agents. Idarucizumab and hemodialysis are not effective for reversal of the parenteral direct thrombin inhibitors, but aPCC or PCC may be utilized if drug cessation alone is insufficient (16,21).
Tissue plasminogen activator (tPA)
tPA cleaves plasminogen to plasmin, a potent fibrinolytic enzyme. It may be administered systemically in select patients with ischemic cerebrovascular accidents, pulmonary embolism, and STEMI; it may also be infused locally for catheter-directed thrombolysis or disruption of loculated pleural effusion (34). Bleeding complications are typically managed by cessation of tPA as the half-life of first generation fibrinolytics such as alteplase is extremely short (35). While there is no specific reversal agent available, anti-fibrinolytics such as ε-aminocaproic acid or tranexamic acid and careful maintenance of adequate fibrinogen level may be used to decrease bleeding severity.
Acquired hemophilia A (AHA)
Acquired inhibitors to coagulation factors are rare. Inhibitors to FVIII, with the resultant clinical presentation commonly referred to as AHA, are the most common with an incidence of one per 1.5 million (36). Acquired hemophilia is caused by polyclonal IgG autoantibodies that bind and neutralize FVIII causing its deficiency (37). Acquired hemophilia may be idiopathic or associated with autoimmune diseases, pregnancy, malignancy, drugs, and infection (36,38). The median age of onset is 64 to 78 years (36,38,39). In contrast to congenital hemophilia A, patients with AHA typically present with soft tissue, muscle, or mucosal bleeding rather than joint bleeding, although presentation is variable. In AHA, prolonged aPTT is likely to correct in the immediate mix with normal plasma, but will remain prolonged after incubation at 37 ℃ due to a time- and temperature-dependent inhibitor (37). Once the FVIII activity level is confirmed to be low, the inhibitor should be quantified in the Bethesda assay (40). Management of AHA is two-fold: control of bleeding and eradication of the inhibitor. Control of hemorrhage is achieved with agents that either increase FVIII or bypass the FVIII inhibitor, with the latter being the most common approach. The benefit of agents that raise FVIII activity, including desmopressin (DDAVP) or FVIII concentrates, is the ability to monitor response with FVIII activity assays, but these interventions are only effective in patients with very low titer inhibitors [≤5 Bethesda Units (BU)] (40,41). Recombinant porcine FVIII (rPFVIII) was found to be 86% effective at controlling bleeding in patients with AHA, but this product requires a dedicated assay for monitoring (42). Agents that bypass FVIII, including rFVIIa and aPCC, are commonly used in the first line setting to treat bleeds associated with AHA in those patients with high titer inhibitors (>5 BU) or life-threatening bleeds. The efficacy of bypassing agents is determined by clinical response and cannot be monitored by aPTT or FVIII activity. Both rFVIIa and aPCC have similar response rates in excess of 90% (41). The use of emicizumab in AHA is an area of active investigation. In a series of 12 patients, emicizumab proved to have hemostatic efficacy, but prospective clinical trials are needed (43).
Acquired hemophilia B (AHB)
AHB occurs less frequently than AHA, with the exact incidence unknown due to a scarcity of reporting. Acquired inhibitors to FIX can occur alone or in conjunction with other factor inhibitors (44,45). Like AHA, patients with AHB typically present with soft tissue or muscle bleeding and are of older age. Identified etiologies include autoimmune diseases, malignancy, drugs, pregnancy, and infection such as viral hepatitis and HIV (46). Control of bleeding should be obtained by using rFVIIa or aPCC (47). Inhibitor eradication has been achieved with immunosuppressants such as prednisone, rituximab, and azathioprine (44,45,48,49).
Disseminated intravascular coagulation (DIC)
DIC or consumptive coagulopathy is characterized by systemic activation of coagulation resulting in fibrin generation and microvascular thrombi leading to organ dysfunction and consumption of clotting factors and platelets (50-53). DIC occurs in about 1% of hospitalized patients and simultaneous occurrence of thrombosis and bleeding complicates the care of these patients. In a majority of them, DIC is secondary to an underlying illness such as severe infection, trauma, surgery, malignancy, obstetric complications, transfusion reactions, vascular abnormalities, fat embolism, toxins or catastrophic antiphospholipid syndrome. Pathogenesis and clinical presentation of acute DIC is different from chronic DIC. While acute DIC presents commonly with bleeding due to consumption of procoagulant factors, chronic DIC does not have a bleeding component because of the compensatory production of coagulation factors and platelets despite the ongoing thrombin generation. As such, laboratory parameters vary in both of these conditions (Table 1). Scoring systems are recommended for the diagnosis of DIC as suggested by several guidelines. The International Society on Thrombosis and Haemostasis (ISTH) developed a practical scoring system for the diagnosis of overt DIC that uses commonly available laboratory tests and requires the presence of an underlying disorder known to be associated with DIC. Global coagulation tests such as the PT and aPTT, fibrinogen, platelet count, evidence of fibrinolysis as measured by the D-dimer level, and blood smear examination provide important information on the degree of coagulation factor activation and consumption. However, there is no specific test to confirm the diagnosis, and these abnormalities may also be seen in individuals with severe liver disease, warfarin treatment, and thrombotic microangiopathy. Decompensated cirrhosis is characterized by accelerated intravascular coagulation and fibrinolysis, termed as AICF; relatively stable platelet count and high FVIII distinguish this process from DIC as does the absence of uncompensated thrombin generation (Table 1). The most important treatment for DIC is controlling the underlying disorder; management is based on consensus recommendations due to paucity of randomized trials to guide therapy (50-53). In general, transfusions are not indicated simply for abnormal laboratory values, and should be reserved for patients with bleeding or at high risk of bleeding or those requiring invasive procedures. In critically ill patients with DIC without bleeding and platelet count >30,000/µL, prophylaxis of venous thromboembolism with heparin or LMWH is often recommended. In patients with severe sepsis, thrombosis and severe purpura fulminans associated with acral ischemia or vascular skin infarction, therapeutic doses of heparin should be considered, due to its short half-life and reversibility in the event of bleeding (50-54). In general, antifibrinolytic agents are contraindicated in DIC due to concern for thrombotic complications. These agents may be appropriate in DIC patients who have severe bleeding associated with a hyperfibrinolytic state such as in trauma. A phase III trial of activated protein C concentrate in patients with sepsis and DIC demonstrated a significant reduction in mortality (55). However, a meta-analysis of published studies found no evidence of benefit and activated protein C concentrate is associated with a higher risk of bleeding (56). The use of antithrombin concentrates has been evaluated in randomized controlled trials in patients with severe sepsis but did not show a reduction in mortality (57). Future randomized studies evaluating novel agents and anticoagulation are warranted to improve outcomes in DIC patients.
Table 1
Laboratory parameters | Liver disease | Acute DIC | Chronic DIC |
---|---|---|---|
Platelet count | ↓↓ – ↓ | ↓↓ – ↓ | Variable |
PT | ↑↑ | ↑↑ – ↔ | ↔ |
aPTT | ↑ – ↔ | ↑↑ – ↔ | ↔ |
Fibrinogen | ↓↓ – ↔ | ↓↓ – ↔ | ↑ – ↔ |
D-dimer | ↑ | ↑↑ | ↑ |
Peripheral smear | Nonspecific, thrombocytopenia | Schistocytes, thrombocytopenia | Schistocytes, thrombocytopenia |
FVIII | ↑ | ↓ | ↔ |
FV | ↓ | ↓ | ↔ |
DIC, disseminated intravascular coagulation; PT, prothrombin time; aPTT, activated partial thromboplastin time; FVIII, factor VIII; FV, factor V.
Liver failure
The hepatocyte is the site of production of thrombopoietin (TPO) and several coagulation factors, including fibrinogen, prothrombin FII, FV, FVII, FIX, FX and FXI, as well as proteins involved in fibrinolysis (58-61). In addition to a synthetic function, post-translational modifications such as glycosylation and gamma-carboxylation of factors also occur in the liver, leading to functional abnormalities in liver disease (58-61). In addition, hepatocytes and Kupffer cells are responsible for clearing activated coagulation factors and products of fibrinolysis from the circulation. Hemostasis in patients with liver dysfunction is rebalanced, owing to the parallel reduction of procoagulant and anticoagulant factors, impacting all stages of coagulation (Table 2) (58-61). In addition to thrombocytopenia from decreased synthesis due to low TPO and splenic sequestration, platelet function abnormalities have also been reported (62,63). Concomitant factors such as severity of cirrhosis, gastrointestinal varices due to portal hypertension, endothelial dysfunction, use of alcohol, vitamin K deficiency, infection, and coexisting renal dysfunction may also influence the risk of bleeding. Conventional tests of the clotting cascade such as the PT and aPTT correlate poorly with procedure-related bleeding in patients with cirrhosis as they only reflect changes in procoagulant factors. However, in critically ill cirrhosis patients, abnormal coagulation parameters and high DIC scores with low fibrinogen and platelets correspond to increased bleeding risk. Viscoelastic testing such as thromboelastography (TEG) or thromboelastometry (ROTEM) are increasingly being used for perioperative transfusion guidance in patients undergoing liver transplantation. TEG-guided blood product transfusion strategy has also been shown to reduce blood product transfusion without increased risk of bleeding in cirrhotic patients undergoing invasive procedures (64-66). Increasing recognition of the rebalanced hemostasis in liver disease has led to changes in prophylactic measures, and current guidelines recommend a restrictive approach to pro-hemostatic therapy in bleeding patients (67,68). It is prudent to evaluate and consider underlying factors such as infections, medications, renal dysfunction, nutritional deficiencies and avoid volume overload to reduce any side-effects on portal pressure and varices. Oral or parenteral vitamin K can be administered to individuals with suspected poor nutrition and cirrhosis, as well as those with cholestasis, diarrhea or antibiotic use. There is no definitive evidence that correction of thrombocytopenia, a prolonged PT, or abnormal TEG or ROTEM tests by infusion of donor plasma, platelet concentrates, or low volume coagulation factor concentrates are effective at achieving rapid hemostasis. Variceal bleeding, which accounts for a high percentage of bleeding episodes overall in individuals with cirrhosis is considered to be predominantly due to increased portal pressure and resulting vascular abnormalities rather than a primary hemostatic defect (67,68). Current guidelines do not recommend plasma transfusion to treat portal hypertensive bleeding or for prophylactic use prior to paracentesis in patients with increased INR (68,69). Restrictive transfusion strategies have been shown to significantly improve outcomes in patients with acute upper gastrointestinal bleeding. Therefore, recent guidance statements have argued against prophylactic correction of hemostasis parameters, and strongly recommend that blood products should be used sparingly in patients with acute or chronic liver disease (67,68). In patients undergoing liver biopsy, rescue of bleeding with rFVIIa was both safer and less expensive than conventional plasma-based prophylactic strategies (61). Unlike plasma, PCCs and rFVIIa effectively and safely reduced the INR in critically ill patients with coagulopathy associated with liver impairment to expedite interventions. The amount of blood products used was significantly lower in the PCC and rFVIIa groups, possibly reducing the risk of hypervolemia, while bleeding rates were similar across groups (70). Their use in acute variceal bleeding has not been adequately studied and could theoretically increase thrombotic risk. Two TPO receptor agonists are approved for peri-procedural use in patients with chronic liver disease and thrombocytopenia, demonstrating efficacy and safety in increasing platelet count and reducing the need for transfusions (71,72). However, the trials were not designed to assess bleeding risk in thrombocytopenia, and their use is limited by a slow onset of action (5 days or more before platelets rise with a peak of 12–14 days), which requires advanced planning. Antifibrinolytics agents, ε-aminocaproic acid and tranexamic acid may also be considered in patients with hyperfibrinolysis though their efficacy is unproven. Caution is advised when using antifibrinolytic agents in patients with liver disease as they may be at risk for thrombotic complications.
Table 2
Primary hemostasis |
Deceased thrombopoietin |
Thrombocytopenia |
Altered platelet function |
Increased nitric oxide |
Increased prostacyclin |
Coagulation pathway |
Decreased coagulation factor levels |
Dysfibrinogenemia |
Fibrinolysis |
Elevated tPA |
Decreased α2AP and PAI-1 |
Decreased FXIII and TAFI |
Concomitant bleeding conditions |
Portal hypertension/varices |
Medications |
Infections |
Renal dysfunction |
Vitamin K deficiency |
tPA, tissue plasminogen activator; α2AP, alpha 2-antiplasmin; PAI-1, plasminogen activator inhibitor-1; FXIII, Factor XIII; TAFI, thrombin activatable fibrinolysis inhibitor.
Acknowledgments
The authors would like to thank all individuals living with and/or caring for those with disorders of hemostasis.
Funding: None.
Footnote
Provenance and Peer Review: This article was commissioned by the Guest Editor (Paul D. Mintz) for the series “Transfusion Therapy: Principles and Practices” published in Annals of Blood. The article has undergone external peer review.
Conflicts of Interest: All authors have completed the ICMJE uniform disclosure form (available at https://aob.amegroups.com/article/view/10.21037/aob-21-78/coif). The series “Transfusion Therapy: Principles and Practices” was commissioned by the editorial office without any funding or sponsorship. RG reports Frommeyer Fellowship in Investigative Medicine, University of Alabama at Birmingham; National Center for Advancing Translational Sciences of the National Institutes of Health under award number KL2TR003097; Centers for Disease Control and Prevention/DHHS award number, AWD00000288SUB00000138. RG serves as Consultant for Sanofi Genzyme and Alexion. The authors have no other conflicts of interest to declare.
Ethical Statement: The authors are accountable for all aspects of the work in ensuring that questions related to the accuracy or integrity of any part of the work are appropriately investigated and resolved.
Open Access Statement: This is an Open Access article distributed in accordance with the Creative Commons Attribution-NonCommercial-NoDerivs 4.0 International License (CC BY-NC-ND 4.0), which permits the non-commercial replication and distribution of the article with the strict proviso that no changes or edits are made and the original work is properly cited (including links to both the formal publication through the relevant DOI and the license). See: https://creativecommons.org/licenses/by-nc-nd/4.0/.
References
- Srivastava A, Santagostino E, Dougall A, et al. WFH Guidelines for the Management of Hemophilia, 3rd edition. Haemophilia 2020;26 Suppl 6:1-158.
- Iorio A, Stonebraker JS, Chambost H, et al. Establishing the prevalence and prevalence at birth of hemophilia in males: a meta-analytic approach using national registries. Ann Intern Med 2019;171:540-6. [Crossref] [PubMed]
- Manco-Johnson MJ, Abshire TC, Shapiro AD, et al. Prophylaxis versus episodic treatment to prevent joint disease in boys with severe hemophilia. N Engl J Med 2007;357:535-44. [Crossref] [PubMed]
- Gringeri A, Lundin B, von Mackensen S, et al. A randomized clinical trial of prophylaxis in children with hemophilia A (the ESPRIT Study). J Thromb Haemost 2011;9:700-10. [Crossref] [PubMed]
- Godby RC, Raju D, Massicano F, et al. Optimizing factor VIII dosing in obese individuals with haemophilia A. Blood Coagul Fibrinolysis 2021;32:528-30. [Crossref] [PubMed]
- Lewandowska MD, Connors JM, Factor XI. Deficiency. Hematol Oncol Clin North Am 2021;35:1157-69. [Crossref] [PubMed]
- Menegatti M, Peyvandi F. Treatment of rare factor deficiencies other than hemophilia. Blood 2019;133:415-24. [Crossref] [PubMed]
- Reynolds MW, Clark J, Crean S, et al. Risk of bleeding in surgical patients treated with topical bovine thrombin sealants: a review of the literature. Patient Saf Surg 2008;2:5. [Crossref] [PubMed]
- Ragni MV, Lewis JH, Spero JA, et al. Factor VII deficiency. Am J Hematol 1981;10:79-88. [Crossref] [PubMed]
- Ruiz-Saez A. Occurrence of thrombosis in rare bleeding disorders. Semin Thromb Hemost 2013;39:684-92. [Crossref] [PubMed]
- Kerlin BA, Inbal A, Will A, et al. Recombinant factor XIII prophylaxis is safe and effective in young children with congenital factor XIII-A deficiency: international phase 3b trial results. J Thromb Haemost 2017;15:1601-6. [Crossref] [PubMed]
- Menegatti M, Palla R, Boscarino M, et al. Minimal factor XIII activity level to prevent major spontaneous bleeds. J Thromb Haemost 2017;15:1728-36. [Crossref] [PubMed]
- Deaton JG, Nappe TM. Warfarin toxicity 2021.
- Nikolakopoulos I, Spyropoulos AC. Heparin bridging therapy for patients on chronic oral anticoagulants in periprocedural settings. Semin Thromb Hemost 2020;46:26-31. [Crossref] [PubMed]
- Piran S, Schulman S. Treatment of bleeding complications in patients on anticoagulant therapy. Blood 2019;133:425-35. [Crossref] [PubMed]
- Witt DM, Nieuwlaat R, Clark NP, et al. American Society of Hematology 2018 guidelines for management of venous thromboembolism: optimal management of anticoagulation therapy. Blood Adv 2018;2:3257-91. [Crossref] [PubMed]
- Dunois C. Laboratory Monitoring of direct oral anticoagulants (DOACs). Biomedicines 2021;9:445. [Crossref] [PubMed]
- Cuker A, Siegal DM, Crowther MA, et al. Laboratory measurement of the anticoagulant activity of the non-vitamin K oral anticoagulants. J Am Coll Cardiol 2014;64:1128-39. [Crossref] [PubMed]
- Cuker A, Husseinzadeh H. Laboratory measurement of the anticoagulant activity of edoxaban: a systematic review. J Thromb Thrombolysis 2015;39:288-94. [Crossref] [PubMed]
- Schreuder M, Reitsma PH, Bos MHA. Reversal agents for the direct factor xa inhibitors: biochemical mechanisms of current and newly emerging therapies. Semin Thromb Hemost 2020;46:986-98. [Crossref] [PubMed]
- Marano G, Vaglio S, Pupella S, et al. How we treat bleeding associated with direct oral anticoagulants. Blood Transfus 2016;14:465-73. [PubMed]
- Connolly SJ, Crowther M, Eikelboom JW, et al. Full study report of andexanet alfa for bleeding associated with factor Xa inhibitors. N Engl J Med 2019;380:1326-35. [Crossref] [PubMed]
- Onishi A, St Ange K, Dordick JS, et al. Heparin and anticoagulation. Front Biosci (Landmark Ed) 2016;21:1372-92. [Crossref] [PubMed]
- Takemoto CM, Streiff MB, Shermock KM, et al. Activated partial thromboplastin time and anti-xa measurements in heparin monitoring: biochemical basis for discordance. Am J Clin Pathol 2013;139:450-6. [Crossref] [PubMed]
- May JE, Siniard RC, Taylor LJ, et al. From activated partial thromboplastin time to antifactor Xa and back againthe evolution of monitoring unfractionated heparin. Am J Clin Pathol 2022;157:321-27. [Crossref] [PubMed]
- Khor B, Van Cott EM. Laboratory tests for antithrombin deficiency. Am J Hematol 2010;85:947-50. [Crossref] [PubMed]
- Levy JH, Connors JM. Heparin resistance - clinical perspectives and management strategies. N Engl J Med 2021;385:826-32. [Crossref] [PubMed]
- Gray E, Mulloy B, Barrowcliffe TW. Heparin and low-molecular-weight heparin. Thromb Haemost 2008;99:807-18. [Crossref] [PubMed]
- Thomas O, Lybeck E, Strandberg K, et al. Monitoring low molecular weight heparins at therapeutic levels: dose-responses of, and correlations and differences between aPTT, anti-factor Xa and thrombin generation assays. PLoS One 2015;10:e0116835. [Crossref] [PubMed]
- Sokolowska E, Kalaska B, Miklosz J, et al. The toxicology of heparin reversal with protamine: past, present and future. Expert Opin Drug Metab Toxicol 2016;12:897-909. [Crossref] [PubMed]
- van Veen JJ, Maclean RM, Hampton KK, et al. Protamine reversal of low molecular weight heparin: clinically effective? Blood Coagul Fibrinolysis 2011;22:565-70. [Crossref] [PubMed]
- Elmer J, Wittels KA. Emergency reversal of pentasaccharide anticoagulants: a systematic review of the literature. Transfus Med 2012;22:108-15. [Crossref] [PubMed]
- Van Cott EM, Roberts AJ, Dager WE. Laboratory monitoring of parenteral direct thrombin inhibitors. Semin Thromb Hemost 2017;43:270-6. [Crossref] [PubMed]
- Hughes RE, Tadi P, Bollu PC. TPA Therapy 2021.
- Davydov L, Cheng JW. Tenecteplase: a review. Clin Ther 2001;23:982-97; discussion 981. [Crossref] [PubMed]
- Collins PW, Hirsch S, Baglin TP, et al. Acquired hemophilia A in the United Kingdom: a 2-year national surveillance study by the United Kingdom Haemophilia Centre Doctors’ Organisation. Blood 2007;109:1870-7. [Crossref] [PubMed]
- Kershaw G, Favaloro EJ. Laboratory identification of factor inhibitors: an update. Pathology 2012;44:293-302. [Crossref]
- Knoebl P, Marco P, Baudo F, et al. Demographic and clinical data in acquired hemophilia A: results from the European Acquired Haemophilia Registry (EACH2). J Thromb Haemost 2012;10:622-31. [Crossref] [PubMed]
- Delgado J, Jimenez-Yuste V, Hernandez-Navarro F, et al. Acquired haemophilia: review and meta-analysis focused on therapy and prognostic factors. Br J Haematol 2003;121:21-35. [Crossref] [PubMed]
- Ma AD, Carrizosa D. Acquired factor VIII inhibitors: pathophysiology and treatment. Hematology Am Soc Hematol Educ Program 2006;432-7. [Crossref] [PubMed]
- Baudo F, Collins P, Huth-Kühne A, et al. Management of bleeding in acquired hemophilia A: results from the European Acquired Haemophilia (EACH2) Registry. Blood 2012;120:39-46. [Crossref] [PubMed]
- Kruse-Jarres R, St-Louis J, Greist A, et al. Efficacy and safety of OBI-1, an antihaemophilic factor VIII (recombinant), porcine sequence, in subjects with acquired haemophilia A. Haemophilia 2015;21:162-70. [Crossref] [PubMed]
- Knoebl P, Thaler J, Jilma P, et al. Emicizumab for the treatment of acquired hemophilia A. Blood 2021;137:410-9. [Crossref] [PubMed]
- Cui QY, Wu TQ, Shen HS, et al. Refractory lupus nephropathy and acquired Factor VIII and IX deficiencies in a patient with systemic lupus erythematosus treated with rituximab. Haemophilia 2017;23:e504-6. [Crossref] [PubMed]
- Carmassi F, Giannarelli C, De Giorgi A, et al. Combined factor VIII and IX inhibitors in a non-haemophilic patient: successful treatment with immunosuppressive drugs. Haemophilia 2007;13:106-7. [Crossref] [PubMed]
- Páramo L, Enciso Olivera LJ, Noreña I, et al. First case of acquired hemophilia B in a patient with HIV infection: Case Report and Literature Review. Cureus 2019;11:e4179. [Crossref] [PubMed]
- Franchini M, Castaman G, Coppola A, et al. Acquired inhibitors of clotting factors: AICE recommendations for diagnosis and management. Blood Transfus 2015;13:498-513. [PubMed]
- Krishnamurthy P, Hawche C, Evans G, et al. A rare case of an acquired inhibitor to factor IX. Haemophilia 2011;17:712-3. [Crossref] [PubMed]
- Mazzucconi MG, Peraino M, Bizzoni L, et al. Acquired inhibitor against factor IX in a child: successful treatment with high-dose immunoglobulin and dexamethasone. Haemophilia 1999;5:132-4. [PubMed]
- Levi M. Current understanding of disseminated intravascular coagulation. Br J Haematol 2004;124:567-76. [Crossref] [PubMed]
- Levi M, Sivapalaratnam S. Disseminated intravascular coagulation: an update on pathogenesis and diagnosis. Expert Rev Hematol 2018;11:663-72. [Crossref] [PubMed]
- Levi M, Toh CH, Thachil J, et al. Guidelines for the diagnosis and management of disseminated intravascular coagulation. British Committee for Standards in Haematology. Br J Haematol 2009;145:24-33. [Crossref] [PubMed]
- Wada H, Thachil J, Di Nisio M, et al. Guidance for diagnosis and treatment of DIC from harmonization of the recommendations from three guidelines. J Thromb Haemost 2013; [Crossref] [PubMed]
- Levi M, Scully M. How I treat disseminated intravascular coagulation. Blood 2018;131:845-54. [Crossref] [PubMed]
- Bernard GR, Vincent JL, Laterre PF, et al. Efficacy and safety of recombinant human activated protein C for severe sepsis. N Engl J Med 2001;344:699-709. [Crossref] [PubMed]
- Martí-Carvajal AJ, Solà I, Gluud C, et al. Human recombinant protein C for severe sepsis and septic shock in adult and paediatric patients. Cochrane Database Syst Rev 2012;12:CD004388. [Crossref] [PubMed]
- Warren BL, Eid A, Singer P, et al. Caring for the critically ill patient. High-dose antithrombin III in severe sepsis: a randomized controlled trial. JAMA 2001;286:1869-78. [Crossref] [PubMed]
- Drolz A, Ferlitsch A, Fuhrmann V. Management of Coagulopathy during Bleeding and Invasive Procedures in Patients with Liver Failure. Visc Med 2018;34:254-8. [Crossref] [PubMed]
- Lisman T, Leebeek FW, de Groot PG. Haemostatic abnormalities in patients with liver disease. J Hepatol 2002;37:280-7. [Crossref] [PubMed]
- Tripodi A, Mannucci PM. The coagulopathy of chronic liver disease. N Engl J Med 2011;365:147-56. [Crossref] [PubMed]
- Caldwell SH, Hoffman M, Lisman T, et al. Coagulation disorders and hemostasis in liver disease: pathophysiology and critical assessment of current management. Hepatology 2006;44:1039-46. [Crossref] [PubMed]
- Laffi G, Marra F, Gresele P, et al. Evidence for a storage pool defect in platelets from cirrhotic patients with defective aggregation. Gastroenterology 1992;103:641-6. [Crossref] [PubMed]
- Ordinas A, Escolar G, Cirera I, et al. Existence of a platelet-adhesion defect in patients with cirrhosis independent of hematocrit: studies under flow conditions. Hepatology 1996;24:1137-42. [Crossref] [PubMed]
- Leon-Justel A, Noval-Padillo JA, Alvarez-Rios AI, et al. Point-of-care haemostasis monitoring during liver transplantation reduces transfusion requirements and improves patient outcome. Clin Chim Acta 2015;446:277-83. [Crossref] [PubMed]
- Coakley M, Reddy K, Mackie I, et al. Transfusion triggers in orthotopic liver transplantation: a comparison of the thromboelastometry analyzer, the thromboelastogram, and conventional coagulation tests. J Cardiothorac Vasc Anesth 2006;20:548-53. [Crossref] [PubMed]
- Wang SC, Shieh JF, Chang KY, et al. Thromboelastography-guided transfusion decreases intraoperative blood transfusion during orthotopic liver transplantation: randomized clinical trial. Transplant Proc 2010;42:2590-3. [Crossref] [PubMed]
- Northup PG, Lisman T, Roberts LN. Treatment of bleeding in patients with liver disease. J Thromb Haemost 2021;19:1644-52. [Crossref] [PubMed]
- Northup PG, Garcia-Pagan JC, Garcia-Tsao G, et al. Vascular liver disorders, portal vein thrombosis, and procedural bleeding in patients with liver disease: 2020 practice guidance by the American Association for the study of liver diseases. Hepatology 2021;73:366-413. [Crossref] [PubMed]
- Garcia-Tsao G, Abraldes JG, Berzigotti A, et al. Portal hypertensive bleeding in cirrhosis: Risk stratification, diagnosis, and management: 2016 practice guidance by the American Association for the study of liver diseases. Hepatology 2017;65:310-35. [Crossref] [PubMed]
- Kwon JO, MacLaren R. Comparison of fresh-frozen plasma, four-factor prothrombin complex concentrates, and recombinant factor VIIa to facilitate procedures in critically Ill patients with coagulopathy from liver disease: A Retrospective Cohort Study. Pharmacotherapy 2016;36:1047-54. [Crossref] [PubMed]
- Terrault N, Chen YC, Izumi N, et al. Avatrombopag before procedures reduces need for platelet transfusion in patients with chronic liver disease and thrombocytopenia. Gastroenterology 2018;155:705-18. [Crossref] [PubMed]
- Peck-Radosavljevic M, Simon K, Iacobellis A, et al. Lusutrombopag for the treatment of thrombocytopenia in patients with chronic liver disease undergoing invasive procedures (L-PLUS 2). Hepatology 2019;70:1336-48. [Crossref] [PubMed]
Cite this article as: Godby RC, Gangaraju R, May JE, Kommalapati A, Paschal R, Marques MB. Congenital and acquired disorders of secondary hemostasis. Ann Blood 2022;7:11.