Fetal and neonatal immune thrombocytopenia caused by maternal alloantibodies and isoantibodies in Caucasian and Asian populations: a narrative review
Human platelet antigens (HPAs) associated with fetal neonatal and alloimmune thrombocytopenia (FNAIT)
Common HPAs
Alloantigens
Twelve well-established HPAs are included in six diallelic systems: HPA-1, HPA-2, HPA-3, HPA-4, HPA-5 and HPA-15. HPAs are numbered in their order of discovery, with the higher frequency antigen designated as ‘a’ and the lower frequency antigen designated as ‘b’. The lower frequency antigens arose from single nucleotide mutations on the respective gene leading to missense amino acid exchanges and resulting in new antigenic determinants (epitopes). The frequencies of HPAs were intensively studied in Caucasian populations, and investigated in other ethnic groups.
Nonself antigens causing immune responses in members of the same species are called alloantigens or isoantigens. Alloantigens (such as HPA) differ only in the polymorphic structure caused by mutational changes (e.g., single amino acid substitutions) of glycoprotein, and isoantigens are recognized by individuals lacking an entire cellular glycoprotein present in cells of normal individuals. Accordingly, the corresponding immune responses are called alloimmunization or isoimmunization. Until today, isoantigens have not been included in the HPA nomenclature.
Three transmembrane glycoprotein complexes, namely, αIIbβ3, α2β1, and GPIb/IX/V, and a glycosylphosphatidylinositol (GPI)-linked glycoprotein, CD109, are known as carriers of antigenic determinants. HPA-1, HPA-3, and HPA-4 reside on αIIbβ3, whereas HPA-5 resides on α2β1, and HPA-15 is found on CD109. In the last three decades, a large number of rare HPAs have been identified. This progress is due in particular to the introduction of a platelet crossmatch between maternal serum and paternal platelets (carrier of rare antigens) in the antigen capture assay (MAIPA), in addition to antibody screening of maternal serum using standard platelet panels. As a result, the first low-frequency HPA (HPA-8b) could be discovered (1). Most of them are located on the extracellular domain of the integrin αIIbβ3 (https://www.versiti.org/medical-professionals/precision-medicine-expertise/platelet-antigen-database/hpa-gene-database).
Alloantibodies and FNAIT
In this section, we will discuss the recent progress on the most clinically important human platelet antigens, namely, HPA-1, -3, and -4 residing on integrins αIIbβ3, and HPA-5 on α2β1 (Figure 1A,1B) as well as HPA-2 and HPA-15 located on GPIb/IX/V complex and CD109, respectively. We present the following article in accordance with the Narrative Review reporting checklist (available at https://dx.doi.org/10.21037/aob-21-47).
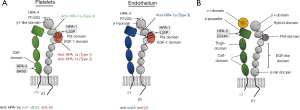
HPA-1 and HPA-4 on β3 integrin
HPA-1 is the most relevant alloantigen system associated with FNAIT in Caucasians. Alloantibodies against HPA-1a are responsible for approximately 80% of FNAIT cases (4,5). The incidence of FNAIT is about 1:1,000 in newborns (6). In a retrospective study, intracranial hemorrhage (ICH) was encountered in approximately 15% of these cases (4), which may lead to fetal death or persistent neurological sequelae (7). In a prospectively studied cohort of pregnancies, the incidence of ICH was lower. In 79 newborn babies with FNAIT (anti-HPA-1a), 2 (2.5%) developed ICH. In both cases, platelet counts were <50×109/L (8). Among Caucasian populations, the frequency of the rare HPA-1b allele ranges from 0.133 to 0.190. In contrast, HPA-1b gene frequencies are much lower in East Asian populations (ranging from 0.002 to 0.012) (9). Accordingly, alloimmunization against HPA-1a is unlikely in Asian populations. Recently, the first case of FNAIT due to anti-HPA-1a antibodies was found in a blood-related Chinese family (10).
HPA-1a and -1b are controlled by the point mutation leucine > proline at position 33 (Leu33Pro) located on the PSI (plexins, semaphorins and integrins) domain of the β3 integrin subunit (Figure 1A). According to the allele distribution, the estimated risk of an antigenic incompatibility allowing immunization against HPA-1b is higher compared to HPA-1a (13.51% versus 2.17% for a first pregnancy). However, alloimmunization against HPA-1a is much more common than immunization against HPA-1b during pregnancy. In addition, only a few cases of FNAIT caused by anti-HPA-1b were reported (11), whereas, anti-HPA-1b antibodies are encountered more frequently than anti-HPA-1a in patients immunized by blood transfusion. Until today, the reason for this discrepancy is unclear.
Although the Leu33Pro mutation responsible for the formation of HPA-1a and -1b could be identified, little is known about the exact epitopes recognized by anti-HPA-1a antibodies. Accumulated data demonstrated that the epitopes of anti-HPA-1a antibodies are heterogeneous. Some anti-HPA-1a react solely with the PSI domain carrying the polymorphic amino acid residue 33 (called type 1), and some recognize conformational epitopes formed by the PSI-together with the epidermal growth factor-1 (EGF-1) domain (called type 2) (2,12-14) (Figure 1A). Recent studies showed that both antibody types bind independently from the α subunits (neither αIIb nor αv) (15). Furthermore, current evidence indicated that most anti-HPA-1a represent type 2 antibodies (13). Therefore, it is tempting to speculate that type 1 and type 2 antibodies represent successive affinity maturation stages during the course of the immune response (13). A study demonstrated that anti-HPA-1a antibodies are also heterogeneous in their ability to interfere with fibrinogen binding and consequently have a different impact on bleeding in FNAIT (16). It is currently unclear whether this functional heterogeneity is related to type 1 and type 2 anti-HPA-1a antibodies.
It is known that the integrin β3 common subunits can form heterodimers with either αIIb or αv chains and function as a receptor for fibrinogen (αIIbβ3) and vitronectin (αvβ3), respectively (Figure 1A). Recently, another type of anti-HPA-1a antibody recognizing compound epitopes formed by the αv, and the polymorphic β3 subunit was found in FNAIT cases, predominantly found in FNAIT cases with ICH. However, the exact position and conformation of binding sites of this antibody type are not known. In comparison to the previous antibody types, this anti-HPA-1a type could alter angiogenesis (3). Similar observations were made in an animal model suggesting that maternal anti-β3 antibodies suppressed fetal angiogenesis and caused trophoblasts destruction by natural killer cells leading to ICH, placental dysfunction and miscarriage (17,18). It remains to be elucidated which types of anti-β3 antibodies produced by immunized mice in this animal model are directly responsible for this dysfunction.
Recent observations indicated that anti-HPA-1a antibodies recognizing the αvβ3 complex did not significantly induce platelet clearance by macrophages, most probably due to the low copy number of αvβ3 on the platelet surface when compared to αIIbβ3 (500 versus 50,000 molecules/platelet) (Santoso et al., in preparation). HPA-1a antibodies have been shown to suppress megakaryopoiesis by inducing early cell death (19). Later, Zeng et al. demonstrated that anti-αvβ3 autoantibodies from patients with immune thrombocytopenia (ITP) could aggravate thrombocytopenia by impeding megakaryocytes migration and adhesion to the vascular niche (20). Thus, maternal anti-HPA-1a reacting specifically with fetal αvβ3 may alter fetal/neonatal platelet counts by inhibiting thrombocytopoiesis.
Finally, although the three-dimensional structures of the isolated PSI domain in αvβ3 and αIIbβ3 are nearly superimposable, this domain showed distinct conformational states when placed in the context of the entire β3 pairing either with αv or αIIb subunit. This structural difference could be recognized by the immune system (3). Therefore, it is possible that different patterns of anti-HPA-1 antibody types may develop by immunization with platelets or endothelial cells.
Recent data indicated that maternal immunization to HPA-1a occurs during pregnancy by exposure to β3 on syncytiotrophoblast or platelets (Figure 2). In addition, immunization by fetal platelets may occur from obstetrical, trauma complications, or at parturition (6). As HPA-1a is expressed on αvβ3 integrin in the fetal trophoblast, one might speculate that contact of maternal blood with trophoblast or circulating microparticles in maternal blood result in maternal alloimmunization against HPA-1a on β3 and αvβ3 complex (21-23). In addition, if immunization occurs to HPA-1a fetal platelets residing on αIIbβ3, anti-HPA-1a antibodies against β3 and αIIbβ3 complex may develop (Figure 1A).
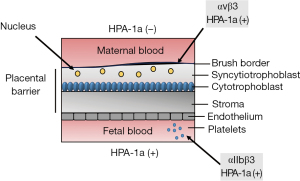
In the Japanese population, anti-HPA-4b is the most frequent maternal αIIbβ3 antibody in FNAIT (24). The allelic frequency of HPA-4b among the Japanese is 0.00854, whereas the HPA-4 polymorphism is nearly nonexistent in Caucasian populations. Surprisingly, two FNAIT cases caused by anti-HPA-4b have been reported in Caucasian families (25,26). HPA-4a and -4b are formed by the point mutation arginine > glutamine at position 143 located on the β I-domain of the β3 integrin subunit (Figure 1A). Currently, it is unknown whether different types of anti-HPA-4 antibodies recognizing different epitopes exist.
HPA-3 on αIIb integrin
In comparison to HPA-1, a greater variation of HPA-3 frequencies was found among different populations. The frequency of HPA-3a and HPA-3b ranges from 0.50 to 0.61 and 0.39 to 0.50, respectively (27). Despite these balanced allele frequencies, anti-HPA-3 antibodies are rarely detected in the sera of women with thrombocytopenic newborns. However, ICH related to anti-HPA-3 antibodies was reported (28-32). Several serological studies showed that anti-HPA-3 antibodies are difficult to detect, most probably because of the heterogeneity of HPA-3 epitopes (33).
HPA-3a and -3b result from the single amino acid Ile843Ser substitution located near the carboxyl terminus of the αIIb extracellular domain (34). Early biochemical studies identified serine 837 as a major O-glycosylation site required for anti-HPA-3a antibody binding (35) (Figure 1A). In this respect, some anti-HPA-3 alloantibodies required intact epitopes formed by O-linked carbohydrates structures or terminal sialic acids that can be lost during platelet storage and platelets’ isolation process for antibody testing (28,29,36-38). A similar phenomenon was observed with the rare alloantigen HPA-9 (Val837Met) located six amino acids away from the HPA-3 dimorphism (39). Recently, a rare platelet antigen on αIIb (HPA-30bw) associated with FNAIT was reported. This antigen is formed by the point mutation Glu806His located within the calf-2 domain and also depends on the carbohydrate composition of αIIb subunit. Similar to some anti-HPA-3a, anti-HPA-30bw alloantibodies are sensitive to O-glycanase treatment (40). However, O-glycosylation is regulated differently in non-human, human cell lines, or human platelets, which are likely to express completely different glycan chains. In accordance, anti-HPA-30b antibodies did react with HPA-30b expressed in human (HEK293) cell line but not in non-human (CHO-K1) cells (40). Recently, bioengineered induced pluripotent stem cells (iPSC)-derived megakaryocytes for the detection of HPA-3 and HPA-9bw were generated (41). In the future, the development of in vitro-generated HPA-defined iPSC-derived platelets carrying endogenous carbohydrates moieties may improve the detection of challenging platelet antibodies.
The αIIb and αv subunits of the β3 integrin family are apparently different, although both share significant homology (42). The αIIbβ3 integrin is expressed on platelets but not on endothelial cells (43). Earlier studies demonstrated that anti-HPA-3a did not react with endothelial cells compared to anti-HPA-1a antibodies (44). In the mature protein, the single heavy chain of αIIb (GPIIbα) is disulfide-bonded to the light chain (GPIIbβ). Biochemical studies demonstrated the existence of two GPIIbβ isoforms, which may lead to the dual proteolytic processing of αIIb (45). Whether this phenomenon may alter αIIb expression on different cells and consequently the binding of some anti-HPA-3 antibodies has not been clarified. Although αIIb is claimed to be a platelet-specific integrin, Rout et al. reported high αIIb expression on invasive trophoblast cells in the mice. Together with other integrins, αIIbβ3 coordinately regulate the adhesion and migration of the differentiating mouse trophoblast (46). Ongoing study in animal models using αIIb deficient and human αIIb transgenic mice indicated that maternal anti-αIIb may target trophoblast cells and lead to placenta dysfunction (47).
It is becoming more and more apparent that different antibody types produced as a polyclonal response by the mother during the pregnancy may determine the severity of the disease. Therefore, the development of serological identification and functional analysis of such antibody type is an important area to improve the prediction of severity in FNAIT cases.
HPA-5 on α2 integrin
Anti-HPA-5b antibodies were first identified in the maternal sera of patients with FNAIT (48,49). In Caucasian populations, anti-HPA-5b is the second most frequent alloantibody type (9–19%) encountered with FNAIT (5,50,51). Compared to anti-HPA-1a, anti-HPA-5 antibodies were found in higher frequency in unselected female blood donors with at least one pregnancy screened for platelet antibodies (52). Our data showed that 1.7% and 0.1% of female blood donors with at least one previous pregnancy present anti-HPA-5b and anti-HPA-1a, respectively (53). However, the chance for a thrombocytopenic child in immunized pregnant women with anti-HPA-5b is low. This observation is in accordance with a previous prospective study in a large cohort of 933 pregnant women screened to develop platelet alloantibodies. Seventeen of 933 mothers (1.8%) formed anti-HPA-5b, but in all cases the newborns’ platelet counts were above 150×109/L (54). In a Japanese cohort of FNAIT cases, anti-HPA-5b was also found as the most frequent platelet antibody (24). Although thrombocytopenia in HPA-5b-FNAIT is often moderate, cases with ICH have been observed (55,56). Anti-HPA-5a, directed against the antithetic antigen, has been found in FNAIT and platelet transfusion refractoriness (PTR) (57,58).
HPA-5a and -5b result from the single amino acid substitution Glu534Lys, which is located on the β-propeller-domain of the a2 subunit of the α2β1 integrin (Figure 1B). α2β1, originally characterized on long-term activated T-cells, has been subsequently found on several cell types, including platelets, fibroblast, epithelial cells, and endothelial cells depending on differentiation and activation state. The α2β1 integrin mainly acts as a receptor for collagens, but other non-collagenous proteins, including laminin, proteoglycans, and viruses, have been described as ligands as well (59).
In contrast to αIIbβ3, only a few copies of the α2β1 integrin (2,000–6,000 copies/platelet) were found on platelets. Similar to the α-subunits of β2-integrin, α2 has a 191-amino acid insert (called the I-domain), which is highly homologous to the A-domain found in the von Willebrand factor (VWF) (60). There are several consistent pieces of evidence for the heterogeneity of α2 expression on platelets. First, two spots with different isoelectric points corresponding to α2 integrin subunits were found (61). Then, a different copy number of α2β1 on the platelet surface associated with an α2 gene polymorphism was reported. Individuals carrying the 807T/873A allele express high levels of platelet α2β1 when compared to individuals carrying the 807C/873G allele (62). Further studies showed a linkage between HPA-5b with C807 (low expresser) and HPA-5a with either C807 or T807 (low and high expresser). This observation indicated that some HPA-5a positive individuals expressed α2β1 in high density on the platelet surface. The HPA-5 polymorphism did not alter platelet adhesion onto collagens (63) but attenuated the adhesion of platelets onto decorin, a small leucine-rich proteoglycan (64).
It is conceivable that the expression of HPA-5 on different cells, depending on the surface density, different state and activation of α2β1 integrin, may enhance antibody binding, alter cellular functions leading to severe cases of FNAIT.
Isoantibodies against αIIbβ3 and FNAIT
Isoantibodies against αIIbβ3 produced in mothers with Glanzmann thrombasthenia (GT) are responsible for fetal and neonatal ITP. GT is a rare autosomal recessive disorder of platelet aggregation caused by mutations in the ITGA2B or/and ITGB3 genes encoding the integrin αIIbβ3. Three types of GT based on αIIbβ3 expression/function have been described: type I with absent αIIbβ3 expression (<5% of normal), type II with reduced expression (5–20% of normal), and type III, which has normal expression but is characterized by non-functional αIIbβ3. Recurrent bleeding episodes and platelet immunization are common complications of this disease (65). In rare cases, isoantibodies against αIIbβ3 developed by GT mothers (type I) before or during the pregnancy could cause FNAIT. Such isoantibodies reacted with all platelets derived from healthy blood donors (66-69). A systematic review of the literature among 31 cases comprising 40 pregnancies showed maternal isoimmunization in 73% of pregnancies and was associated with four neonatal deaths (70).
Recently, a unique mutation in the ITGA2B gene (G > A substitution at the splice donor site of intron 15) was found within the French Gypsy that showed a strong linkage to the HPA-1b allele (71). Based on the αIIb defect, these homozygous HPA-1bb Gypsy patients fail to express the αIIbβ3 heterodimer on the platelet surface, but can still express αvβ3 carrying HPA-1b epitopes. Interestingly, these GT patients developed two types of anti-HPA-1a alloantibodies, one that reacted with β3, and another that recognized the αvβ3 complex (14).
Isoantibodies against CD36 in immune-mediated disorders
Three decades ago, a new platelet antigen (designated Naka) involved in PTR was reported for the first time (72,73). By immunochemical analysis, the authors demonstrated that anti-Naka antibodies reacted with platelet membrane glycoprotein IV (GPIV, today designated as CD36) from normal donors but not from Naka negative subjects. Further study showed that platelets from Naka negative platelets failed to express CD36 (CD36null) on the cell surface, suggesting that Naka is an isoantigen rather than an alloantigen. Consequently, only transfusions with Naka negative platelets were able to raise the platelet counts of the patients. Several cases of PTR associated with anti-CD36 antibodies, primarily in Asian and African populations were reported from this time forward. However, PTR cases caused by anti-CD36 were also found in individuals of Mediterranean descent, including Lebanon, Egypt, Oman, and Palestine (74-81).
Meanwhile, several anti-CD36 immune-mediated diseases were described, including thrombotic thrombocytopenic purpura (TTP) (82-84), hemolytic uremic syndrome (HUS) (85), adverse transfusion reactions (86,87), post-transfusion purpura (PTP) (88), and FNAIT (89,90). Interestingly, transfusion-related acute lung injury (TRALI) associated with plasma transfusions containing anti-CD36 antibodies has also been reported (91).
Scavenger receptor CD36
CD36 is a multifunctional membrane receptor highly expressed on vascular and hematopoietic cells, including vascular smooth muscle cells, endothelial cells, macrophages, platelets and fetal erythrocytes. The class B scavenger receptor family, binds to many different ligands, including thrombospondin, oxidized phospholipids, oxidized low-density lipoprotein (oxLDL), and long-chain fatty acids. Furthermore, CD36 also mediates the adhesion of Plasmodium falciparum, Staphylococcus, and Mycobacterium to infected erythrocytes. Accordingly, CD36 is involved in diverse physiological and pathological processes, including thrombosis/hemostasis, atherogenesis, the innate immune defense, and diabetes (92-94).
The human CD36 gene is located on chromosome 7q11.2 and is encoded by 15 exons, but only exons 3 to 14 encode a 472 amino acid peptide [~molecular weight (MW) 50 kDa] (95,96). The protein harbors two transmembrane domains, a large extracellular domain containing ligand-binding sites and a single short cytoplasmic tail at each terminal (N and C terminal). The extracellular domain includes a hydrophobic region between amino acids 184–204 that may interact with the plasma membrane (Figure 3). The extracellular domain is heavily glycosylated; 9/10 putative N-linked glycosylation sites are glycosylated. Different CD36 molecular weights were reported for CD36 isolated from platelets, epithelial cells, and erythroblasts [relative molecular mass (Mr) ranging from 78 to 88 kDa], indicating cell type-specific glycosylation (92). Phylogenetic studies of the CD36 gene among vertebrates, including humans, mice, chickens and zebrafish, indicated that the CD36 gene appeared early in evolution, before the emergency of bony fish more than 500 million years ago. Human and mouse CD36 share high homology (more than 80%), leading to strong similarities in the structure and tissue distribution (97). Accordingly, several monoclonal antibodies (mAbs) against human CD36 showed cross-reactivity with mouse CD36 and vice versa (98).
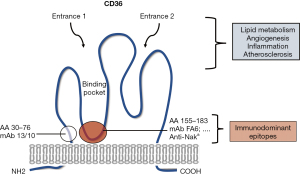
Besides the transmembrane form of CD36, a soluble form of CD36 (sCD36) associated with microparticles was identified. Elevated sCD36 plasma concentration was detected in patients with cardiovascular disease and diabetes (99). However, little is currently known about the function of sCD36. Recent data demonstrated that type I CD36 deficient individuals characterized by the absence of CD36 on platelets and monocytes failed to produce sCD36 in plasma. This allows rapid identification of these subjects by analyzing plasma samples using a solid-phase sandwich ELISA (100).
Immunodominant epitopes on CD36
The first IgG mAb against CD36, designated as FA6-152, was generated by immunizing with fetal erythrocytes. mAb FA6-152 binds to fetal monocytes, platelets, reticulocytes, but not lymphocytes and granulocytes. Interestingly, this mAb agglutinated fetal, but not adult erythrocytes (101). Shortly after that, several mAbs against CD36 were assigned by the International Workshop of Leukocyte Typing (102). Some anti-CD36 mAb (5F1; IgM) lysed platelets in plasma and some (ES IVC7; IgG) induced platelet aggregation, and most of them inhibited collagen-induced platelet aggregation.
Using human-mouse chimeric expressing cells, Daviet et al. could identify two different immunodominant epitopes recognized by mAbs (103). The majority of mAbs, including FA6-152, recognized epitopes within the domain comprising amino acids 155–183. One unique mAb, 13/10, bound another domain that spans amino acids 30–76. MAbs against the 155–183 domain is functionally important regarding their capability to inhibit ligand binding, platelet function, and adhesion of P. falciparum-infected erythrocytes onto CD36 (103,104).
Further studies showed that all mAbs recognizing the 155–183 domain inhibited the binding of anti-CD36 antibodies in human sera, whereas mAb against the 30–76 domain did not. These observations indicate that the 155–183 amino acid sequence is important for the binding of anti-CD36 antibodies (105). Therefore, detection of anti-CD36 by an antigen-capture assay using mAb as capture antibody (such as MAIPA) frequently leads to false-negative results caused by competitive inhibition between anti-CD36 mAb and human serum (106). Thus, the selection of appropriate capture mAb is important to eliminate the drawback of the antigen-capture assay for the identification of anti-CD36 antibodies in serum samples. This fact, however, could be useful for the development of therapeutic antibodies: modified mAbs against CD36 competing for the same binding site could be used to displace the binding of human anti-CD36 antibodies preventing the pathogenic effects of these antibodies (98).
CD36 deficiency
The existence of CD36 deficient (Naka negative) subjects was described for the first time in Japan. Among the Japanese population, around 3–11% of healthy blood donors were Naka negative lacking CD36 expression on the platelet surface (72,107). Further studies analyzing CD36 expression on monocytes showed two different types of CD36 deficiency. Type I individuals are characterized by the absence of CD36 both on platelets and monocytes whereas type II subjects fail to express CD36 on platelets only (108). Two features are unique to the CD36 deficiency. First, the incidence of CD36 deficiency is relatively high as compared with other glycoprotein deficiencies, such αIIbβ3 in GT and GPIb/IX in Bernard-Soulier syndrome (BSS) patients (109,110), who present with hemostatic problems. Second, CD36 deficient individuals are apparently healthy without evidence of hemostatic abnormalities (72,107,111). However, studies in humans and mice showed defective uptake and utilization of long fatty acids, indicating a causal link between defective myocardial fatty acids uptake and CD36 deficiency (94,112).
Population studies have shown that the incidence of CD36 deficiency varies among different ethnic groups. CD36 deficiency is very rare in Caucasians (<0.3%) and is more common in African and Asian populations (4–8%) (74,75,113). A higher frequency of CD36-deficient individuals (type I and type II) was reported in Japan (6.8%) (113). In China, the frequency of CD36 deficiency on platelets ranges from 1.8% to 3.6% in the largest ethnic group, the Han population (81,114,115). Similar frequency (2.64%) was reported in the Arabian population (116).
More than 30 mutations in the coding region of the CD36 gene responsible for type I CD36 deficiency have been described (115). Three common mutations, C268T, 949insA, and 329–330 delAC, have been identified in Japanese individuals (117). Although the C268T mutation represents the most frequent substitution (>50%), this polymorphism did not lead to the production of anti-CD36 isoantibodies in reported cases (Table S1). In contrast, 329–330 delAC and 1,228–1,239 delATTGTGCCTATT are the most common mutations in Chinese individuals (81). In Thailand, A1163T seems to be the most frequent mutation associated with CD36 deficiency (100). Because of these heterogenous mutations, a practicable genotyping approach for identifying individuals with CD36 deficiency is difficult to develop.
Anti-CD36 isoantibodies and FNAIT
Shortly after the discovery of anti-CD36 antibodies as a cause of PTR in Japan (72), the first case of FNAIT due to anti-CD36 antibodies was reported in Thailand (89). After that, the clinical relevance of maternal anti-CD36 antibodies was reinforced by other new cases of FNAIT, three from African-American mothers and one from a mother with Italian ancestry (90). Meanwhile, several FNAIT cases due to anti-CD36 antibodies were identified in China (118). Recently, anti-CD36 associated with FNAIT was found in Arabic populations living in Germany (116). Table S1 provides a synopsis of previously published clinical cases, their diagnostics, and treatments (81,89,90,116,119-124). Serological diagnostics of the CD36 antigen and antibody will be discussed separately in another review chapter.
Pathomechanisms of anti-CD36 mediated FNAIT
Similar to previously known alloantibody-mediated FNAIT cases, the degree of thrombocytopenia caused by anti-CD36 isoantibodies varies widely from moderate to severe. ICH was also reported. Besides thrombocytopenia, fetal anemia frequently accompanies the clinical picture of the fetus.
In contrast to anti-HPA-1a, anti-CD36 antibodies react with platelets and endothelial cells, and also erythrocytes and monocytes of the fetus. Former studies applying mAbs (clones FA6-152 and 5F1) demonstrated the expression of CD36 solely on fetal but not on adult erythrocytes. The CD36 expression was related to the differentiation stage of the erythroid progenitors. It is expressed on mature BFU-E with an antigenic density increasing until day five CFU-E (101). It is well known that antigen-antibody-mediated red cell hemolysis (e.g., against Rhesus D) can cause anemic hydrops fetalis (AHF), leading to widespread soft-tissue edema and/or the accumulation of fluid in the fetal body cavities (125). Several studies reported an association between anti-CD36 antibodies and AHF (119,121,124). Recently, we found AHF caused by anti-CD36 in a case of severe FNAIT. Maternal anti-CD36 antibodies caused a significant reduction of BFU-E/CFU-E formation associated with an increased number of apoptotic CD34+ erythroid/myeloid precursor cells (124). This mechanism may be responsible for fetal anemia and consecutive AHF. Nevertheless, intrauterine transfusion with washed red blood cells (RBCs) should be taken into account to prevent fetal anemia and improve fetal survival (121).
CD36 is found on the placental membranes, microvillus, and basal membrane (126). The central role of CD36 (also known as fatty acid translocase) as a high-affinity receptor for fatty acid uptake and lipid metabolism has been well documented (127,128). During the third trimester of pregnancy, the placenta’s preferential transport of maternal plasma FA is critical for fetal growth and development (129). Disturbance of placenta vascular development and function could dramatically alter fetal growth development and thereby neonatal survival. In this process, placental vascularization and angiogenesis play critical roles (130,131). Recently, we found in the mouse FNAIT model a significant reduction of the placental labyrinth area and decreased fetal capillary numbers in immunized Cd36−/− mothers compared to the naïve cohort. This phenomenon depends on FcγRIIa (CD32) expressed on placenta microvascular endothelial cells (132). It is conceivable that crosslinking between endothelial and blood cells by anti-CD36 antibodies may enhance the inflammatory response leading to severe FNAIT and even fetal death by affecting placenta angiogenesis (98).
Natural history of maternal immunization
Prospective studies on FNAIT in Caucasian populations provided information on the natural history of FNAIT. Cumulative data from ten prospective studies (133) show that 9.7% of all HPA-1a (−) negative mothers are immunized during pregnancy. The chance that a HPA-1a (−) mother gives birth to a HPA-1a (+) child is approximately 84%, given a gene frequency of 0.839 for HPA-1a (134). In the large Norwegian study (8), 34.2% of all HPA-1a (+) neonates of immunized mothers were severely thrombocytopenic (platelet counts <50×109/L), whereas 47.2% had normal counts with platelets >150×109/L. In this study the rate of FNAIT cases with ICH (platelet counts <150×109/L) was 2.4%. This rate may be the consequence of the screening and treatment strategy applied by the investigators: as children of all immunized mothers were delivered 2 to 4 weeks prior to term by cesarean section. Furthermore, HPA-1a immunization is strongly correlated with the HLA-DRB3*01:01 class II major histocompatibility antigen (135). In HPA-1a (−) women giving birth to HPA-1a (+) progeny, the risk of immunization is about 25 times higher if the mothers are a carrier of HLA-DRB3*01:01 (136). Accordingly, the outcome of the neonates is associated with the HLA-DRB3*01:01 (137).
Little is known about the natural history of maternal immunization against other platelet alloantigens and isoantigens until today. Therefore, such a study is important to predict the risk of immunization.
Current therapies of FNAIT
Antenatal prophylactic and postnatal therapeutic interventions aim to reduce the risk of ICH and other serious bleeding complications in the fetus and the newborn. Most therapeutic recommendations and guidelines on prenatal therapy refer to anti-HPA-1a mediated FNAIT. In HPA-1a immunized women with at least one previously affected child, the risk for the next pregnancy depends upon the paternal genotype (HPA-1aa or HPA-1ab). In case of heterozygous father (HPA-1ab) the fetal HPA genotype should be determined, preferably by (noninvasive) typing of cell free fetal DNA in maternal plasma (138-140). The risk of fetal ICH is enhanced, if a previous sibling with FNAIT suffered from ICH (141) and the clinical outcome of the newborn seems to be worse, if the mother carries the DRB3*01:01 antigen (142). For pregnancies at risk, maternal intravenous immunoglobulin (IVIG) therapy (1 g/kg once a week) is considered to be effective in avoiding cerebral bleeding. Due to the lack of randomized studies with a control group, maternal IVIG for preventing fetal ICH in NAIT is used off-label. It is not clear whether additional maternal therapy with corticosteroids is effective (143). In the past, high-risk patients have been subjected to fetal blood sampling and platelet counting followed by intrauterine transfusion of compatible platelets in case of thrombocytopenia. However, owing to the high risk of fetal complications, the non-invasive approach with weekly IVIG infusions is now preferred (143).
Normally, pregnant women in their first pregnancy are not aware of the risk of immunization. Therefore, the screening program for platelet alloimmunization is deliberately performed, but it is still intensively debated. If thrombocytopenic newborns of alloimmunized mothers are at risk of serious bleeding, including ICH, the therapy of choice is the transfusion of antigen-compatible platelets, including maternal platelets (144). However, in urgent situations, platelet concentrates from random donors are usually also effective (144,145). In case platelet concentrates are not available, IVIG may be used (144).
Consensus for standard pre- and postnatal therapies for other antibodies, except HPA-1, is not yet available.
New therapeutic approaches
Although the use of IVIG for the prevention/treatment of FNAIT during pregnancy is increasing, the precise mechanism of IVIG action is still unclear (143). Several mechanisms have been proposed, including decreasing of maternal antibody production by inducing immune tolerance or reduction of placental transfer, and enhancement of maternal IgG catabolism via the FcRn receptor (146-148). However, such strategies may inhibit the transfer of immune-protective maternal IgG, with potential increased risk of infections by the locally dominant pathogens during pregnancy and during the first weeks after birth. Therefore, more specific and effective antenatal therapies are desirable.
The first concept is the development of FcRn inhibitors. Studies in mouse models demonstrated that anti-FcRn antibodies could decrease miscarriages and improve angiogenesis by inhibiting fetal FcRn (17,131,149-151). FcRn is a pH-dependent FcγR that only binds IgG at acidic pH (152). Following pinocytosis into endosomes of endothelial cells, IgG binds to FcRn (due to acidic milieu) and thereby escapes rapid degradation. The FcRn-IgG complex can be translocated back to the cell surface, and IgG can be released after dissociation from FcRn (due to neutral milieu). This recycling process is responsible for the relatively long half-life of IgG. Consequently, inhibition by anti-FcRn leads to rapid IgG degradation and clearance from the circulation (152). This effect reduces anti-platelet antibodies, which has been shown in ITP patients treated with high-dose IVIG (153). Thus, anti-FcRn antibodies may have two modes of action in FNAIT; reduction of maternal antibody concentration and inhibition of FcRn-mediated transfer via the placenta into the fetus (154).
Currently, a clinical trial with the inhibitory anti-FcRn blocking mAb nipocalimab (M281) has been initiated for the therapy of hemolytic disease of the fetus and newborn (HDFN). Therefore, it seems warranted to consider this therapy for FNAIT when this approach turns out to be effective for the therapy of HDFN (154).
An attractive alternative strategy would be to administer non-destructive IgG, which shares the allospecificity of maternal antibodies and can inhibit the binding of pathogenic maternal alloantibody to fetal platelets. Such a therapeutic agent should not trigger effector function by inability to interact with fetal FcγRs, but still retain the ability to be transported across the placenta. Ghevaert et al. introduced such a strategy by developing human recombinant anti-HPA-1a (termed B2G1Δnab) (155). These modified mAb against HPA-1a would be an excellent candidate for treatment of FNAIT if it could be shown in humans that it is able to cross the placenta in sufficient amounts and can displace effectively bound to fetal platelets (155).
In recent years, the therapeutic potential of deglycosylated IgG (deg-IgG) antibodies for treatment of autoimmune disorders has been widely recognized (156). Removal of the N-glycan (linked to asparagine 297), located on the Fc part, leads to a significant reduction of IgG binding with the FcγRs expressed on macrophages and its ability to activate complement C1q. Interestingly, these deg-IgG antibodies could still be transported from the maternal circulation to the fetus via FcRn (157). Accordingly, our previous in vivo study in mice demonstrated that deg-mAb specific for HPA-1a (mAb SZ21) could cross the placenta and prevent the clearance of fetal platelets mediated by maternal anti-HPA-1a antibodies. This observation indicates that the use of epitope-specific antibodies for the antenatal therapy of severe FNAIT is feasible (158).
Recently, we evaluated this treatment strategy in mice model of anti-CD36-mediated FNAIT. By the antenatal administration (5 mg/kg body weight) of deglycosylated mouse mAb 32-106 (deg-32-106), the high frequency of fetal death could be significantly reduced (40% to 2%) However, similar antenatal treatment with IVIG administered in a dose of 1 g/kg body weight on days 10, 15, and 20 after breeding did not increase platelet counts. Furthermore, it did not reduce fetal death rates (40%). Only IVIG administration three days earlier at days 7, 12, and 17 instead at days 10, 15, and 20 reduced fetal death (40% to 13%) (98). Our results indicated that deg-32-106 could prevent placenta angiogenesis caused by maternal antibodies (98). Interestingly, deg-32-106 reacted with human CD36. Thus, the development of humanized deg-32-106 as a drug to prevent severe FNAIT caused by anti-CD36 could be envisaged.
Another strategy to prevent fetal bleeding complications is to reduce the risk of alloimmunization by administering antibodies to eliminate fetal platelets and fetal cellular particles from the maternal circulation. This has been attempted by administration of anti-HPA-1a antibodies (159). It is assumed that anti-D causes rapid clearance of D positive fetal RBCs from the maternal circulation (160). Tiller et al. were able to transfer this situation of antibody-mediated immune suppression (AMIS) to a mouse model for FNAIT: they reduced the immunizing effects of human platelet transfusions to β3−/− mice with infusions of human anti-HPA-1a and with infusions of mouse monoclonal anti-β3 antibodies (161). First results from a clinical trial show (EudraCT number 2019-003459-12) show that NAITgam is able to reduce the half life of HPA-1a positive platelets transfused to HPA-1a-negative subjects (162). However, more trials are mandatory to show both safety and efficiency of this prophylactic therapy.
Other HPAs
HPA-2 on GPIbα
Immunization against antigens of the biallelic HPA-2 system residing on platelet GPIb/IX/V complex can lead to the production of anti-HPA-2a and anti-HPA-2b alloantibodies. The HPA-2 polymorphism, discovered in Caucasians, also exists in other Asian populations (118) and in sub-Saharan African populations (163). The rarer allele, HPA-2b, has a frequency of 0.088 in the Western European population (134). In Han Chinese, the frequency of this antigen is 0.0485 and in Koreans 0.077 (118). In sub-Saharan populations, HPA-2b frequencies vary from 0.224 to 0.393 (163). Alloantibodies against HPA-2b were first discovered in a Dutch patient, who had received blood transfusions (164). Later anti-HPA-2b antibodies were found in a multi-transfused Japanese patient (165). These antibodies have been also found in rare cases of FNAIT (166-168). An atypical case of neonatal amegakaryocytic thrombocytopenia associated with anti-HPA-2b was reported (169).
The antigenic determinants of HPA-2a and HPA-2b are formed by substitution of threonine into methionine at amino acid position 145 located on the amino terminal domain of GPIbα subunit (170). The GPIbα contains a N-terminal ligand binding domain (LBD), an anionic and sulfated region, a macroglycopeptide region and a mechanosensory domain (MSD) (171). This Thr145Met dimorphism resides in the LBD domain within VWF and alters their binding (172). However, little is known about the impact of anti-HPA-2 antibodies on platelet function. Platelet GPIbα is sensitive for protease cleavage (173). A soluble extracellular fragment of GPIbα (glycocalicin) containing anti-HPA-2 binding sites is continuously released due to proteolytic cleavage by ADAM17 metalloproteinase (171). This fact may hamper the detection of anti-HPA-2 antibodies and may explain the rarity of these antibodies.
The glycoprotein GPIb-IX-V complex is expressed exclusively on platelets and megakaryocytes. After integrin αIIbβ3, GPIb/IX/V complex is the second most abundant receptor on human platelets (171). Early study reported that human endothelial cells in culture also expressed GPIb/IX/V complex (174). In contrast to β3 integrin carrying HPA-1a epitopes (22,175), GPIb/X/V complex is not found on trophoblast. One might speculate that this is reason for the rare immunization against HPA-2 system in FNAIT.
HPA-15 on CD109
Immunization against the biallelic HPA-15 system residing on GPI-anchored CD109 glycoprotein can lead to the production of anti-HPA-15a and anti-HPA-15b alloantibodies. These alloantibodies were found in different clinical settings of immune mediated thrombocytopenia including PTR and FNAIT (176-180).
The gene frequencies of HPA-15a and -15b were 0.512 and 0.488 in Caucasians (181), while 0.532 and 0.468 in Asian populations (182,183). Although the gene frequencies of HPA-15a and HPA-15b are similar, anti-HPA-15b was found more frequently than anti-HPA-15a both in PTR and FNAIT cases (51,180,181). The reason for this phenomenon is currently unknown.
Anti-HPA-15 antibodies were originally discovered by radioimmunoprecipitation (176). Meanwhile, anti-HPA-15 can also be detected using antigen capture assay (184). This approach, however, seems to be insensitive when platelets are used for testing due to the low copy number of CD109 on platelets. The use of cell lines stably expressing high CD109 copy number could reduce the rate of false negative result obtained with platelets (185).
CD109 is expressed in fetal and adult CD34 positive bone marrow cell subsets, activated T lymphoblast, activated platelets, endothelium, megakaryoblastic cell leukemia cells, and human tumor cell lines (186-189). Upregulation of CD109 was observed in several tumor cells (190,191). Cuppini and coworkers reported that CD109 positive circulating endothelial cells was associated with increased progression free survival and overall survival suggesting the role CD109 on the mechanism of tumor progression (192).
On platelets, CD109 is not constitutively expressed on the cell surface, but appears as early activation marker. After agonist-induced activation, such as low-dose ADP and epinephrine, CD109 is rapidly displayed on the platelet surface. In resting platelets, CD109 resides in α-granules, co-distributed with P-selectin and within the open canicular system (OPS). Accordingly, only low expression level of CD109 (500–2,000 molecules/platelet) was detectable on the platelet surface (193). In addition, marked inter-individual differences in the copy number of CD109 were observed on platelets (184,194). However, the physiological role of this differential expression is still unknown.
Since CD109 is a platelet activation antigen, it is remarkable that anti-HPA-15 antibodies, although rare, could also be found in FNAIT cases. Among 213 patients with FNAIT only two (0.94%) were related to anti-HPA-15 antibodies (181). In the same study, HPA-15 antibodies were much more common in sera of transfused patients (27.7%). Interestingly, ICH associated with anti-HPA-15b antibodies has been reported (51,178,184). Currently, little is known about the function of anti-CD109 antibodies. Initial studies demonstrated that CD109 antibodies abrogated responder cell proliferation in a mixed lymphocyte response assay (195). Recently, Ye and coworkers reported that knockdown of CD109 suppressed cell migration resulted in reduction of endothelial tube formation (196). The question whether anti-HPA-15 bound to CD109 on endothelial cells could inhibit cell migration and suppress endothelial tube formation is currently not known.
Conclusions and perspectives
Apart from the well-known platelet alloantibodies, isoantibodies against platelet glycoproteins play an important role in the pathomechanism of FNAIT, particularly anti-CD36 antibodies. Although they seem to be uncommon in the Caucasian population, anti-CD36 isoantibodies are of clinical importance in Asian and other populations. Meanwhile, in the course of world globalization, anti-CD36 mediated FNAIT has been reported in Western countries.
Platelet alloantibodies and isoantibodies not only bind to platelets, as confirmed previously, but also interact with endothelial cells (such as anti-HPA-1a) as well as other blood cells (such as anti-CD36). All these antibody-mediated effects can trigger FNAIT by different mechanisms leading to different clinical pictures and consequently require adapted treatment strategies. For example, the IVIG standard therapy for anti-HPA-1a does not seem effective for anti-CD36, at least in the animal model.
In addition, recent evidence indicated that maternal antibodies are also heterogeneous with respect to their epitopes, and their ratio may differ from one case to other, reflecting the various clinical pictures of FNAIT. Therefore, further improvement on laboratory diagnostics, both on serological and functional analysis of platelet antibodies, is mandatory for the better prediction and management of FNAIT.
To achieve this goal, a further understanding of the nature of the pathogenic antibodies will be necessary. Concerning anti-CD36 antibodies, visible progress has been made in the last few years. However, there are several open questions, namely, on the incidence, natural history, antibody titer/types, severity/clinical pictures in FNAIT, and the involvement of anti-CD36 in other antibody-mediated platelet disorders and TRALI.
Acknowledgments
XZX is a PhD candidate at the University of Giessen, Germany. This work is submitted in partial fulfillment of the requirements of the PhD.
Funding: This work was supported in part by grants from the National Natural Science Foundation of China (81601451 and 81970169).
Footnote
Provenance and Peer Review: This article was commissioned by the Guest Editor (Brian R. Curtis) for the series “Thrombocytopenia Due to Immunization Against CD36” published in Annals of Blood. The article has undergone external peer review.
Reporting Checklist: The authors have completed the Narrative Review reporting checklist. Available at https://dx.doi.org/10.21037/aob-21-47
Peer Review File: Available at https://dx.doi.org/10.21037/aob-21-47
Conflicts of Interest: The authors have completed the ICMJE uniform disclosure form (available at https://dx.doi.org/10.21037/aob-21-47). The series “Thrombocytopenia Due to Immunization Against CD36” was commissioned by the editorial office without any funding or sponsorship. The authors have no other conflicts of interest to declare.
Ethical Statement: The authors are accountable for all aspects of the work in ensuring that questions related to the accuracy or integrity of any part of the work are appropriately investigated and resolved.
Open Access Statement: This is an Open Access article distributed in accordance with the Creative Commons Attribution-NonCommercial-NoDerivs 4.0 International License (CC BY-NC-ND 4.0), which permits the non-commercial replication and distribution of the article with the strict proviso that no changes or edits are made and the original work is properly cited (including links to both the formal publication through the relevant DOI and the license). See: https://creativecommons.org/licenses/by-nc-nd/4.0/.
References
- Santoso S, Kalb R, Kroll H, et al. A point mutation leads to an unpaired cysteine residue and a molecular weight polymorphism of a functional platelet beta 3 integrin subunit. The Sra alloantigen system of GPIIIa. J Biol Chem 1994;269:8439-44. [Crossref] [PubMed]
- Zhi H, Ahlen MT, Thinn AMM, et al. High-resolution mapping of the polyclonal immune response to the human platelet alloantigen HPA-1a (PlA1). Blood Adv 2018;2:3001-11. [Crossref] [PubMed]
- Santoso S, Wihadmadyatami H, Bakchoul T, et al. Antiendothelial αvβ3 Antibodies Are a Major Cause of Intracranial Bleeding in Fetal/Neonatal Alloimmune Thrombocytopenia. Arterioscler Thromb Vasc Biol 2016;36:1517-24. [Crossref] [PubMed]
- Mueller-Eckhardt C, Kiefel V, Grubert A, et al. 348 cases of suspected neonatal alloimmune thrombocytopenia. Lancet 1989;1:363-6. [Crossref] [PubMed]
- Davoren A, Curtis BR, Aster RH, et al. Human platelet antigen-specific alloantibodies implicated in 1162 cases of neonatal alloimmune thrombocytopenia. Transfusion 2004;44:1220-5. [Crossref] [PubMed]
- Curtis BR. Recent progress in understanding the pathogenesis of fetal and neonatal alloimmune thrombocytopenia. Br J Haematol 2015;171:671-82. [Crossref] [PubMed]
- Dale ST, Coleman LT. Neonatal alloimmune thrombocytopenia: antenatal and postnatal imaging findings in the pediatric brain. AJNR Am J Neuroradiol 2002;23:1457-65. [PubMed]
- Kjeldsen-Kragh J, Killie MK, Tomter G, et al. A screening and intervention program aimed to reduce mortality and serious morbidity associated with severe neonatal alloimmune thrombocytopenia. Blood 2007;110:833-9. [Crossref] [PubMed]
- Tan JY, Lian LH, Nadarajan VS. Genetic polymorphisms of human platelet antigens-1 to -6, and -15 in the Malaysian population. Blood Transfus 2012;10:368-76. [PubMed]
- Armawai MI, Yahya NM, Hasan A, et al. The first case of fetal neonatal alloimmune thrombocytopenia caused by anti-HPA-1a antibodies in Asian population. ISBT Sci Ser 2015;10:52-6. [Crossref]
- Winters JL, Jennings CD, Desai NS, et al. Neonatal alloimmune thrombocytopenia due to anti-HPA-1b (PLA2)(Zwb). A case report and review. Vox Sang 1998;74:256-9. [Crossref] [PubMed]
- Thinn AMM, Wang Z, Zhou D, et al. Autonomous conformational regulation of β3 integrin and the conformation-dependent property of HPA-1a alloantibodies. Proc Natl Acad Sci U S A 2018;115:E9105-14. [Crossref] [PubMed]
- Holzwarth ST, Bayat B, Zhu J, et al. Naturally occurring point mutation Cys460Trp located in the I-EGF1 domain of integrin β3 alters the binding of some anti-HPA-1a antibodies. Transfusion 2020;60:2097-107. [Crossref] [PubMed]
- Fiore M, Bayat B, Phuangtham R, et al. Immunization against αIIb β3 and αv β3 in Glanzmann thrombasthenia patients carrying the French Gypsy mutation. J Thromb Haemost 2021;19:255-61. [Crossref] [PubMed]
- Bayat B, Traum A, Berghöfer H, et al. Current Anti-HPA-1a Standard Antibodies React with the β3 Integrin Subunit but not with αIIbβ3 and αvβ3 Complexes. Thromb Haemost 2019;119:1807-15. [Crossref] [PubMed]
- Kroll H, Penke G, Santoso S. Functional heterogeneity of alloantibodies against the human platelet antigen (HPA)-1a. Thromb Haemost 2005;94:1224-9. [Crossref] [PubMed]
- Chen ZY, Oswald BE, Sullivan JA, et al. Platelet physiology and immunology: pathogenesis and treatment of classical and non-classical fetal and neonatal alloimmune thrombocytopenia. Ann Blood 2019;4:29. [Crossref]
- Yougbaré I, Lang S, Yang H, et al. Maternal anti-platelet β3 integrins impair angiogenesis and cause intracranial hemorrhage. J Clin Invest 2015;125:1545-56. [Crossref] [PubMed]
- Liu ZJ, Bussel JB, Lakkaraja M, et al. Suppression of in vitro megakaryopoiesis by maternal sera containing anti-HPA-1a antibodies. Blood 2015;126:1234-6. [Crossref] [PubMed]
- Zeng DF, Chen F, Wang S, et al. Autoantibody against integrin αv β3 contributes to thrombocytopenia by blocking the migration and adhesion of megakaryocytes. J Thromb Haemost 2018;16:1843-56. [Crossref] [PubMed]
- Vanderpuye OA, Labarrere CA, McIntyre JA. A vitronectin-receptor-related molecule in human placental brush border membranes. Biochem J 1991;280:9-17. [Crossref] [PubMed]
- Kumpel BM, Sibley K, Jackson DJ, et al. Ultrastructural localization of glycoprotein IIIa (GPIIIa, beta 3 integrin) on placental syncytiotrophoblast microvilli: implications for platelet alloimmunization during pregnancy. Transfusion 2008;48:2077-86. [Crossref] [PubMed]
- Huppertz B, Kingdom J, Caniggia I, et al. Hypoxia favours necrotic versus apoptotic shedding of placental syncytiotrophoblast into the maternal circulation. Placenta 2003;24:181-90. [Crossref] [PubMed]
- Ohto H, Miura S, Ariga H, et al. The natural history of maternal immunization against foetal platelet alloantigens. Transfus Med 2004;14:399-408. [Crossref] [PubMed]
- Morel-Kopp MC, Blanchard B, Kiefel V, et al. Anti-HPA-4b (anti-Yuk(a)) neonatal alloimmune thrombocytopenia: first report in a Caucasian family. Transfus Med 1992;2:273-6. [Crossref] [PubMed]
- Puig N, Muñiz-Diaz E, Monteagudo E, et al. A second case of neonatal alloimmune thrombocytopenia by anti-HPA-4b (anti-Yuka) in a Caucasian family. Transfus Med 1993;3:164-5. [Crossref] [PubMed]
- Wiwanitkit V. Gene frequencies of the human platelet antigen-3 in different populations. Clin Appl Thromb Hemost 2005;11:89-93. [Crossref] [PubMed]
- Harrison CR, Curtis BR, McFarland JG, et al. Severe neonatal alloimmune thrombocytopenia caused by antibodies to human platelet antigen 3a (Baka) detectable only in whole platelet assays. Transfusion 2003;43:1398-402. [Crossref] [PubMed]
- Lin M, Shieh SH, Liang DC, et al. Neonatal alloimmune thrombocytopenia in Taiwan due to an antibody against a labile component of HPA-3a (Baka). Vox Sang 1995;69:336-40. [PubMed]
- Boehlen F, Kaplan C, de Moerloose P. Severe neonatal alloimmune thrombocytopenia due to anti-HPA-3a. Vox Sang 1998;74:201-4. [Crossref] [PubMed]
- Glade-Bender J, McFarland JG, Kaplan C, et al. Anti-HPA-3A induces severe neonatal alloimmune thrombocytopenia. J Pediatr 2001;138:862-7. [Crossref] [PubMed]
- Kataoka S, Kobayashi H, Chiba K, et al. Neonatal alloimmune thrombocytopenia due to an antibody against a labile component of human platelet antigen-3b (Bak b). Transfus Med 2004;14:419-23. [Crossref] [PubMed]
- Socher I, Zwingel C, Santoso S, et al. Heterogeneity of HPA-3 alloantibodies: consequences for the diagnosis of alloimmune thrombocytopenic syndromes. Transfusion 2008;48:463-72. [Crossref] [PubMed]
- Lyman S, Aster RH, Visentin GP, et al. Polymorphism of human platelet membrane glycoprotein IIb associated with the Baka/Bakb alloantigen system. Blood 1990;75:2343-8. [Crossref] [PubMed]
- Calvete JJ, Muñiz-Diaz E. Localization of an O-glycosylation site in the alpha-subunit of the human platelet integrin GPIIb/IIIa involved in Baka (HPA-3a) alloantigen expression. FEBS Lett 1993;328:30-4. [Crossref] [PubMed]
- Take H, Tomiyama Y, Shibata Y, et al. Demonstration of the heterogeneity of epitopes of the platelet-specific alloantigen, Baka. Br J Haematol 1990;76:395-400. [Crossref] [PubMed]
- Djaffar I, Vilette D, Pidard D, et al. Human platelet antigen 3 (HPA-3): localization of the determinant of the alloantibody Lek(a) (HPA-3a) to the C-terminus of platelet glycoprotein IIb heavy chain and contribution of O-linked carbohydrates. Thromb Haemost 1993;69:485-9. [Crossref] [PubMed]
- Barba P, Pallarés P, Nogués N, et al. Post-transfusion purpura caused by anti-HPA-3a antibodies that are only detectable using whole platelets in the platelet immunofluorescence test. Transfus Med 2010;20:200-2. [Crossref] [PubMed]
- Noris P, Simsek S, de Bruijne-Admiraal LG, et al. Max(a), a new low-frequency platelet-specific antigen localized on glycoprotein IIb, is associated with neonatal alloimmune thrombocytopenia. Blood 1995;86:1019-26. [Crossref] [PubMed]
- Wihadmadyatami H, Heidinger K, Röder L, et al. Alloantibody against new platelet alloantigen (Lap(a)) on glycoprotein IIb is responsible for a case of fetal and neonatal alloimmune thrombocytopenia. Transfusion 2015;55:2920-9. [Crossref] [PubMed]
- Zhang N, Santoso S, Aster RH, et al. Bioengineered iPSC-derived megakaryocytes for the detection of platelet-specific patient alloantibodies. Blood 2019;134:e1-8. [Crossref] [PubMed]
- Fitzgerald LA, Poncz M, Steiner B, et al. Comparison of cDNA-derived protein sequences of the human fibronectin and vitronectin receptor alpha-subunits and platelet glycoprotein IIb. Biochemistry 1987;26:8158-65. [Crossref] [PubMed]
- Kieffer N, Phillips DR. Platelet membrane glycoproteins: functions in cellular interactions. Annu Rev Cell Biol 1990;6:329-57. [Crossref] [PubMed]
- Giltay JC, Leeksma OC, von dem Borne AE, et al. Alloantigenic composition of the endothelial vitronectin receptor. Blood 1988;72:230-3. [Crossref] [PubMed]
- Calvete JJ, Schäfer W, Henschen A, et al. Characterization of the beta-chain N-terminus heterogeneity and the alpha-chain C-terminus of human platelet GPIIb. Posttranslational cleavage sites. FEBS Lett 1990;272:37-40. [Crossref] [PubMed]
- Rout UK, Wang J, Paria BC, et al. Alpha5beta1, alphaVbeta3 and the platelet-associated integrin alphaIIbbeta3 coordinately regulate adhesion and migration of differentiating mouse trophoblast cells. Dev Biol 2004;268:135-51. [Crossref] [PubMed]
- Vadasz B. Pathogenesis of Anti-integrin αIIb-mediated Fetal and Neonatal Alloimmune Thrombocytopenia: Establishment of Novel Murine Models in αIIb Deficient and Human αIIb Transgenic Mice. Master Thesis. Department of Laboratory Medicine and Pathobiology University of Toronto, 2015. Available online: https://tspace.library.utoronto.ca/bitstream/1807/69706/1/Vadasz_Brian_201506_MSc_thesis.pdf
- Kiefel V, Santoso S, Katzmann B, et al. A new platelet-specific alloantigen Bra. Report of 4 cases with neonatal alloimmune thrombocytopenia. Vox Sang 1988;54:101-6. [PubMed]
- Santoso S, Kiefel V, Mueller-Eckhardt C. Immunochemical characterization of the new platelet alloantigen system Bra/Brb. Br J Haematol 1989;72:191-8. [Crossref] [PubMed]
- Kroll H, Kiefel V, Santoso S. Clinical aspects and typing of platelet alloantigens. Vox Sang 1998;74:345-54. [Crossref] [PubMed]
- Ghevaert C, Campbell K, Walton J, et al. Management and outcome of 200 cases of fetomaternal alloimmune thrombocytopenia. Transfusion 2007;47:901-10. [Crossref] [PubMed]
- Schnaidt M, Wernet D. Platelet-specific antibodies in female blood donors after pregnancy. Transfus Med 2000;10:77-80. [Crossref] [PubMed]
- Twilfert M, Sumnig A, Bakchoul T, et al. Abstract IMH-V14: Prevalence of platelet alloantibodies in female blood donors with previous pregnancies. Transfusion Med Hemother 2014;41:29.
- Panzer S, Auerbach L, Cechova E, et al. Maternal alloimmunization against fetal platelet antigens: a prospective study. Br J Haematol 1995;90:655-60. [Crossref] [PubMed]
- Kaplan C, Morel-Kopp MC, Kroll H, et al. HPA-5b (Br(a)) neonatal alloimmune thrombocytopenia: clinical and immunological analysis of 39 cases. Br J Haematol 1991;78:425-9. [Crossref] [PubMed]
- Tiller H, Kamphuis MM, Flodmark O, et al. Fetal intracranial haemorrhages caused by fetal and neonatal alloimmune thrombocytopenia: an observational cohort study of 43 cases from an international multicentre registry. BMJ Open 2013;3:002490. [Crossref] [PubMed]
- Kiefel V, Shechter Y, Atias D, et al. Neonatal alloimmune thrombocytopenia due to anti-Brb (HPA-5a). Report of three cases in two families. Vox Sang 1991;60:244-5. [PubMed]
- Arber C, Bertrand G, Halter J, et al. Platelet refractoriness due to combined anti-HLA and anti-HPA-5a alloantibodies: clinical management during myeloablative allogeneic HSCT and development of a quantitative MAIPA assay. Br J Haematol 2007;139:159-61. [Crossref] [PubMed]
- Madamanchi A, Santoro SA, Zutter MM. α2β1 Integrin. Adv Exp Med Biol 2014;819:41-60. [Crossref] [PubMed]
- Takada Y, Hemler ME. The primary structure of the VLA-2/collagen receptor alpha 2 subunit (platelet GPIa): homology to other integrins and the presence of a possible collagen-binding domain. J Cell Biol 1989;109:397-407. [Crossref] [PubMed]
- Kehrel B, Kokott R, Balleisen L, et al. Selective staining of platelet glycoproteins using two-dimensional O'Farrell gel electrophoresis and avidin-biotin-conjugated lectins. Thromb Haemost 1987;58:960-3. [Crossref] [PubMed]
- Kritzik M, Savage B, Nugent DJ, et al. Nucleotide polymorphisms in the alpha2 gene define multiple alleles that are associated with differences in platelet alpha2 beta1 density. Blood 1998;92:2382-8. [Crossref] [PubMed]
- Santoso S, Kalb R, Walka M, et al. The human platelet alloantigens Br(a) and Brb are associated with a single amino acid polymorphism on glycoprotein Ia (integrin subunit alpha 2). J Clin Invest 1993;92:2427-32. [Crossref] [PubMed]
- Kunicki TJ, Williams SA, Diaz D, et al. Platelet adhesion to decorin but not collagen I correlates with the integrin α2 dimorphism E534K, the basis of the human platelet alloantigen (HPA)-5 system. Haematologica 2012;97:692-5. [Crossref] [PubMed]
- Botero JP, Lee K, Branchford BR, et al. Glanzmann thrombasthenia: genetic basis and clinical correlates. Haematologica 2020;105:888-94. [Crossref] [PubMed]
- Kiefel V, Meyer E, Greinacher A, et al. Neonatal isoimmune thrombocytopenia by anti-GP IIb/IIIa. Beitr Infusionsther 1992;30:450-3. [PubMed]
- Léticée N, Kaplan C, Lémery D. Pregnancy in mother with Glanzmann's thrombasthenia and isoantibody against GPIIb-IIIa: Is there a foetal risk? Eur J Obstet Gynecol Reprod Biol 2005;121:139-42. [Crossref] [PubMed]
- Wijemanne A, Watt-Coote I, Austin S. Glanzmann thrombasthenia in pregnancy: Optimising maternal and fetal outcomes. Obstet Med 2016;9:169-70. [Crossref] [PubMed]
- Barg AA, Hauschner H, Luboshitz J, et al. From thrombasthenia to next generation thrombocytopenia: Neonatal alloimmune thrombocytopenia induced by maternal Glanzmann thrombasthenia. Pediatr Blood Cancer 2018;65:e27376. [Crossref] [PubMed]
- Siddiq S, Clark A, Mumford A. A systematic review of the management and outcomes of pregnancy in Glanzmann thrombasthenia. Haemophilia 2011;17:e858-69. [Crossref] [PubMed]
- Jacquelin B, Tuleja E, Kunicki TJ, et al. Analysis of platelet membrane glycoprotein polymorphisms in Glanzmann thrombasthenia showed the French gypsy mutation in the alphaIIb gene to be strongly linked to the HPA-1b polymorphism in beta3. J Thromb Haemost 2003;1:573-5. [Crossref] [PubMed]
- Ikeda H, Mitani T, Ohnuma M, et al. A new platelet-specific antigen, Naka, involved in the refractoriness of HLA-matched platelet transfusion. Vox Sang 1989;57:213-7. [Crossref] [PubMed]
- Tomiyama Y, Take H, Ikeda H, et al. Identification of the platelet-specific alloantigen, Naka, on platelet membrane glycoprotein IV. Blood 1990;75:684-7. [Crossref] [PubMed]
- Curtis BR, Aster RH. Incidence of the Nak(a)-negative platelet phenotype in African Americans is similar to that of Asians. Transfusion 1996;36:331-4. [Crossref] [PubMed]
- Lee K, Godeau B, Fromont P, et al. CD36 deficiency is frequent and can cause platelet immunization in Africans. Transfusion 1999;39:873-9. [Crossref] [PubMed]
- Fujino H, Ohta K, Taniue J, et al. Primary refractoriness to platelet transfusion caused by Nak(a) antibody alone. Vox Sang 2001;81:42-4. [Crossref] [PubMed]
- Ogata T, Ohto H, Yasuda H, et al. CD36 (Naka) sensitization with platelet-transfusion refractoriness in a liver transplant recipient. Transplantation 2005;79:620. [Crossref] [PubMed]
- Flesch B, Miller J, Repp R, et al. Successful autologous hematopoietic progenitor cell transplantation in a patient with an isoantibody against CD36 (glycoprotein IV, Naka). Bone Marrow Transplant 2008;42:489-91. [Crossref] [PubMed]
- Saw CL, Szykoluk H, Curtis BR, et al. Two cases of platelet transfusion refractoriness associated with anti-CD36. Transfusion 2010;50:2638-42. [Crossref] [PubMed]
- Yin XL, Shen WD, Chen YS, et al. Refractory platelet transfusion in a patient with CD36 deficiency due to pseudothrombocytopenia. Platelets 2011;22:237-40. [Crossref] [PubMed]
- Xu X, Ye X, Xia W, et al. Studies on CD36 deficiency in South China: Two cases demonstrating the clinical impact of anti-CD36 antibodies. Thromb Haemost 2013;110:1199-206. [Crossref] [PubMed]
- Tandon NN, Rock G, Jamieson GA. Anti-CD36 antibodies in thrombotic thrombocytopenic purpura. Br J Haematol 1994;88:816-25. [Crossref] [PubMed]
- Rock G, Chauhan K, Jamieson GA, et al. Anti-CD36 antibodies in patients with lupus anticoagulant and thrombotic complications. Br J Haematol 1994;88:878-80. [Crossref] [PubMed]
- Schultz DR, Arnold PI, Jy W, et al. Anti-CD36 autoantibodies in thrombotic thrombocytopenic purpura and other thrombotic disorders: identification of an 85 kD form of CD36 as a target antigen. Br J Haematol 1998;103:849-57. [Crossref] [PubMed]
- Rock G, Clark W, Sternbach M, et al. Haemolytic uraemic syndrome is an immune-mediated disease: role of anti-CD36 antibodies. Br J Haematol 2005;131:247-52. [Crossref] [PubMed]
- Wakamoto S, Fujihara M, Urushibara N, et al. Heterogeneity of platelet responsiveness to anti-CD36 in plasma associated with adverse transfusion reactions. Vox Sang 2005;88:41-51. [Crossref] [PubMed]
- Morishita K, Wakamoto S, Miyazaki T, et al. Life-threatening adverse reaction followed by thrombocytopenia after passive transfusion of fresh frozen plasma containing anti-CD36 (Nak) isoantibody. Transfusion 2005;45:803-6. [Crossref] [PubMed]
- Bierling P, Godeau B, Fromont P, et al. Posttransfusion purpura-like syndrome associated with CD36 (Naka) isoimmunization. Transfusion 1995;35:777-82. [Crossref] [PubMed]
- Kankirawatana S, Kupatawintu P, Juji T, et al. Neonatal alloimmune thrombocytopenia due to anti-Nak(a). Transfusion 2001;41:375-7. [Crossref] [PubMed]
- Curtis BR, Ali S, Glazier AM, et al. Isoimmunization against CD36 (glycoprotein IV): description of four cases of neonatal isoimmune thrombocytopenia and brief review of the literature. Transfusion 2002;42:1173-9. [Crossref] [PubMed]
- Nakajima F, Nishimura M, Hashimoto S, et al. Role of anti-Nak(a) antibody, monocytes and platelets in the development of transfusion-related acute lung injury. Vox Sang 2008;95:318-23. [Crossref] [PubMed]
- Greenwalt DE, Lipsky RH, Ockenhouse CF, et al. Membrane glycoprotein CD36: a review of its roles in adherence, signal transduction, and transfusion medicine. Blood 1992;80:1105-15. [Crossref] [PubMed]
- Febbraio M, Hajjar DP, Silverstein RL. CD36: a class B scavenger receptor involved in angiogenesis, atherosclerosis, inflammation, and lipid metabolism. J Clin Invest 2001;108:785-91. [Crossref] [PubMed]
- Pepino MY, Kuda O, Samovski D, et al. Structure-function of CD36 and importance of fatty acid signal transduction in fat metabolism. Annu Rev Nutr 2014;34:281-303. [Crossref] [PubMed]
- Armesilla AL, Vega MA. Structural organization of the gene for human CD36 glycoprotein. J Biol Chem 1994;269:18985-91. [Crossref] [PubMed]
- Abumrad NA, el-Maghrabi MR, Amri EZ, et al. Cloning of a rat adipocyte membrane protein implicated in binding or transport of long-chain fatty acids that is induced during preadipocyte differentiation. Homology with human CD36. J Biol Chem 1993;268:17665-8. [Crossref] [PubMed]
- Holmes RS. Comparative Studies of Vertebrate Platelet Glycoprotein 4 (CD36). Biomolecules 2012;2:389-414. [Crossref] [PubMed]
- Xu X, Chen D, Ye X, et al. Successful prenatal therapy for anti-CD36-mediated severe FNAIT by deglycosylated antibodies in a novel murine model. Blood 2021;138:1757-67. [Crossref] [PubMed]
- Little KM, Smalley DM, Harthun NL, et al. The plasma microparticle proteome. Semin Thromb Hemost 2010;36:845-56. [Crossref] [PubMed]
- Phuangtham R, Santoso S, Leelayuwat C, et al. Frequency of CD36 deficiency in Thais analyzed by quantification of CD36 on cell surfaces and in plasma. Transfusion 2020;60:847-54. [Crossref] [PubMed]
- Edelman P, Vinci G, Villeval JL, et al. A monoclonal antibody against an erythrocyte ontogenic antigen identifies fetal and adult erythroid progenitors. Blood 1986;67:56-63. [Crossref] [PubMed]
- Von dem Borne AEGK, Modderman P. Cluster Report: CD36. In: Knapp W, Dörken B, Gilks WR, et al. editors. Leukocyte Typing IV. White cell differentiation antigens. Oxford: Oxford University Press, 1989:996.
- Daviet L, Buckland R, Puente Navazo MD, et al. Identification of an immunodominant functional domain on human CD36 antigen using human-mouse chimaeric proteins and homologue-replacement mutagenesis. Biochem J 1995;305:221-4. [Crossref] [PubMed]
- Daviet L, Craig AG, McGregor L, et al. Characterization of two vaccinia CD36 recombinant-virus-generated monoclonal antibodies (10/5, 13/10): effects on malarial cytoadherence and platelet functions. Eur J Biochem 1997;243:344-9. [Crossref] [PubMed]
- Daviet L, Morel-Kopp MC, Kaplan C, et al. A structural/functional domain on human CD36 is involved in the binding of anti-Nak(a) antibodies. Thromb Haemost 1995;73:543-5. [Crossref] [PubMed]
- Morel-Kopp MC, Daviet L, McGregor J, et al. Drawbacks of the MAIPA technique in characterising human antiplatelet antibodies. Blood Coagul Fibrinolysis 1996;7:144-6. [Crossref] [PubMed]
- Shibata Y, Kim N, Morita S, et al. Monoclonal antibody OKM5 and platelet alloantibody anti-Naka have the same specificity. Proc Japan Acad 1990;66:41-2. [Crossref]
- Yamamoto N, Akamatsu N, Sakuraba H, et al. Platelet glycoprotein IV (CD36) deficiency is associated with the absence (type I) or the presence (type II) of glycoprotein IV on monocytes. Blood 1994;83:392-7. [Crossref] [PubMed]
- Nurden AT. Glanzmann thrombasthenia. Orphanet J Rare Dis 2006;1:10. [Crossref] [PubMed]
- Savoia A, Kunishima S, De Rocco D, et al. Spectrum of the mutations in Bernard-Soulier syndrome. Hum Mutat 2014;35:1033-45. [Crossref] [PubMed]
- Yamamoto N, Akamatsu N, Yamazaki H, et al. Normal aggregations of glycoprotein IV (CD36)-deficient platelets from seven healthy Japanese donors. Br J Haematol 1992;81:86-92. [Crossref] [PubMed]
- Coburn CT, Knapp FF Jr, Febbraio M, et al. Defective uptake and utilization of long chain fatty acids in muscle and adipose tissues of CD36 knockout mice. J Biol Chem 2000;275:32523-9. [Crossref] [PubMed]
- Yanai H, Chiba H, Fujiwara H, et al. Phenotype-genotype correlation in CD36 deficiency types I and II. Thromb Haemost 2000;84:436-41. [Crossref] [PubMed]
- Xu X, Liu Y, Hong X, et al. Variants of CD36 gene and their association with CD36 protein expression in platelets. Blood Transfus 2014;12:557-64. [PubMed]
- Li R, Qiao Z, Ling B, et al. Incidence and molecular basis of CD36 deficiency in Shanghai population. Transfusion 2015;55:666-73. [Crossref] [PubMed]
- Flesch BK, Scherer V, Opitz A, et al. Platelet CD36 deficiency is present in 2.6% of Arabian individuals and can cause NAIT and platelet refractoriness. Transfusion 2021;61:1932-42. [Crossref] [PubMed]
- Kashiwagi H, Tomiyama Y, Nozaki S, et al. Analyses of genetic abnormalities in type I CD36 deficiency in Japan: identification and cell biological characterization of two novel mutations that cause CD36 deficiency in man. Hum Genet 2001;108:459-66. [Crossref] [PubMed]
- Wu G, Zhou Y, Li L, et al. Platelet Immunology in China: Research and Clinical Applications. Transfus Med Rev 2017;31:118-25. [Crossref] [PubMed]
- Okajima S, Cho K, Chiba H, et al. Two sibling cases of hydrops fetalis due to alloimmune anti-CD36 (Nak a) antibody. Thromb Haemost 2006;95:267-71. [Crossref] [PubMed]
- Taketani T, Ito K, Mishima S, et al. Neonatal isoimmune thrombocytopenia caused by type I CD36 deficiency having novel splicing isoforms of the CD36 gene. Eur J Haematol 2008;81:70-4. [Crossref] [PubMed]
- Xu X, Li L, Xia W, et al. Successful management of a hydropic fetus with severe anemia and thrombocytopenia caused by anti-CD36 antibody. Int J Hematol 2018;107:251-6. [Crossref] [PubMed]
- Lin M, Xu X, Lee HL, et al. Fetal/neonatal alloimmune thrombocytopenia due to anti-CD36 antibodies: antibody evaluations by CD36-transfected cell lines. Transfusion 2018;58:189-95. [Crossref] [PubMed]
- Bertrand G, Renac V, Lefaix MC, et al. Neonatal intracranial hemorrhage with a dramatic outcome due to maternal anti CD36 antibodies. Reports 2019;27:7. [Crossref]
- Wu Y, Chen D, Xu X, et al. Hydrops fetalis associated with anti-CD36 antibodies in fetal and neonatal alloimmune thrombocytopenia: Possible underlying mechanism. Transfus Med 2020;30:361-8. [Crossref] [PubMed]
- de Haan TR, Oepkes D, Beersma MFC, et al. Aetiology, diagnosis and treatment of hydrops fetalis. Curr Pediatr Rev 2005;1:63-72. [Crossref]
- Duttaroy AK. Transport of fatty acids across the human placenta: a review. Prog Lipid Res 2009;48:52-61. [Crossref] [PubMed]
- Abumrad NA, Davidson NO. Role of the gut in lipid homeostasis. Physiol Rev 2012;92:1061-85. [Crossref] [PubMed]
- Podrez EA, Byzova TV, Febbraio M, et al. Platelet CD36 links hyperlipidemia, oxidant stress and a prothrombotic phenotype. Nat Med 2007;13:1086-95. [Crossref] [PubMed]
- Duttaroy AK, Basak S. Maternal dietary fatty acids and their roles in human placental development. Prostaglandins Leukot Essent Fatty Acids 2020;155:102080. [Crossref] [PubMed]
- Reynolds LP, Redmer DA. Angiogenesis in the placenta. Biol Reprod 2001;64:1033-40. [Crossref] [PubMed]
- Yougbaré I, Tai WS, Zdravic D, et al. Activated NK cells cause placental dysfunction and miscarriages in fetal alloimmune thrombocytopenia. Nat Commun 2017;8:224. [Crossref] [PubMed]
- Gröger M, Sarmay G, Fiebiger E, et al. Dermal microvascular endothelial cells express CD32 receptors in vivo and in vitro. J Immunol 1996;156:1549-56. [PubMed]
- Kamphuis MM, Paridaans N, Porcelijn L, et al. Screening in pregnancy for fetal or neonatal alloimmune thrombocytopenia: systematic review. BJOG 2010;117:1335-43. [Crossref] [PubMed]
- Vorholt SM, Hamker N, Sparka H, et al. High-Throughput Screening of Blood Donors for Twelve Human Platelet Antigen Systems Using Next-Generation Sequencing Reveals Detection of Rare Polymorphisms and Two Novel Protein-Changing Variants. Transfus Med Hemother 2020;47:33-44. [Crossref] [PubMed]
- Valentin N, Vergracht A, Bignon JD, et al. HLA-DRw52a is involved in alloimmunization against PL-A1 antigen. Hum Immunol 1990;27:73-9. [Crossref] [PubMed]
- Kjeldsen-Kragh J, Olsen KJ. Risk of HPA-1a-immunization in HPA-1a-negative women after giving birth to an HPA-1a-positive child. Transfusion 2019;59:1344-52. [Crossref] [PubMed]
- Kjeldsen-Kragh J, Titze TL, Lie BA, et al. HLA-DRB3*01:01 exhibits a dose-dependent impact on HPA-1a antibody levels in HPA-1a-immunized women. Blood Adv 2019;3:945-51. [Crossref] [PubMed]
- Wienzek-Lischka S, Krautwurst A, Fröhner V, et al. Noninvasive fetal genotyping of human platelet antigen-1a using targeted massively parallel sequencing. Transfusion 2015;55:1538-44. [Crossref] [PubMed]
- Nogués N. Recent advances in non-invasive fetal HPA-1a typing. Transfus Apher Sci 2020;59:102708. [Crossref] [PubMed]
- Ouzegdouh Mammasse Y, Chenet C, Drubay D, et al. A new efficient tool for non-invasive diagnosis of fetomaternal platelet antigen incompatibility. Br J Haematol 2020;190:787-98. [Crossref] [PubMed]
- Radder CM, Brand A, Kanhai HH. Will it ever be possible to balance the risk of intracranial haemorrhage in fetal or neonatal alloimmune thrombocytopenia against the risk of treatment strategies to prevent it? Vox Sang 2003;84:318-25. [Crossref] [PubMed]
- Kjeldsen-Kragh J, Ahlen MT. Foetal and neonatal alloimmune thrombocytopenia - The role of the HLA-DRB3*01:01 allele for HPA-1a-immunisation and foetal/neonatal outcome. Transfus Apher Sci 2020;59:102707. [Crossref] [PubMed]
- Winkelhorst D, Murphy MF, Greinacher A, et al. Antenatal management in fetal and neonatal alloimmune thrombocytopenia: a systematic review. Blood 2017;129:1538-47. [Crossref] [PubMed]
- Lieberman L, Greinacher A, Murphy MF, et al. Fetal and neonatal alloimmune thrombocytopenia: recommendations for evidence-based practice, an international approach. Br J Haematol 2019;185:549-62. [Crossref] [PubMed]
- Kiefel V, Bassler D, Kroll H, et al. Antigen-positive platelet transfusion in neonatal alloimmune thrombocytopenia (NAIT). Blood 2006;107:3761-3. [Crossref] [PubMed]
- Liu P, Li L, Fan P, et al. High-dose of intravenous immunoglobulin modulates immune tolerance in premature infants. BMC Pediatr 2018;18:74. [Crossref] [PubMed]
- Wabnitz H, Khan R, Lazarus AH. The use of IVIg in fetal and neonatal alloimmune thrombocytopenia- Principles and mechanisms. Transfus Apher Sci 2020;59:102710. [Crossref] [PubMed]
- Hansen RJ, Balthasar JP. Intravenous immunoglobulin mediates an increase in anti-platelet antibody clearance via the FcRn receptor. Thromb Haemost 2002;88:898-9. [Crossref] [PubMed]
- Zdravic D, Yougbare I, Vadasz B, et al. Fetal and neonatal alloimmune thrombocytopenia. Semin Fetal Neonatal Med 2016;21:19-27. [Crossref] [PubMed]
- Chen P, Li C, Lang S, et al. Animal model of fetal and neonatal immune thrombocytopenia: role of neonatal Fc receptor in the pathogenesis and therapy. Blood 2010;116:3660-8. [Crossref] [PubMed]
- Li C, Piran S, Chen P, et al. The maternal immune response to fetal platelet GPIbα causes frequent miscarriage in mice that can be prevented by intravenous IgG and anti-FcRn therapies. J Clin Invest 2011;121:4537-47. [Crossref] [PubMed]
- Roopenian DC, Akilesh S. FcRn: the neonatal Fc receptor comes of age. Nat Rev Immunol 2007;7:715-25. [Crossref] [PubMed]
- Ling LE, Hillson JL, Tiessen RG, et al. M281, an Anti-FcRn Antibody: Pharmacodynamics, Pharmacokinetics, and Safety Across the Full Range of IgG Reduction in a First-in-Human Study. Clin Pharmacol Ther 2019;105:1031-9. [Crossref] [PubMed]
- Bussel JB, Vander Haar EL, Berkowitz RL. New developments in fetal and neonatal alloimmune thrombocytopenia. Am J Obstet Gynecol 2021;225:120-7. [Crossref] [PubMed]
- Ghevaert C, Herbert N, Hawkins L, et al. Recombinant HPA-1a antibody therapy for treatment of fetomaternal alloimmune thrombocytopenia: proof of principle in human volunteers. Blood 2013;122:313-20. [Crossref] [PubMed]
- Crispin M. Therapeutic potential of deglycosylated antibodies. Proc Natl Acad Sci U S A 2013;110:10059-60. [Crossref] [PubMed]
- Nimmerjahn F, Ravetch JV. Fcgamma receptors as regulators of immune responses. Nat Rev Immunol 2008;8:34-47. [Crossref] [PubMed]
- Bakchoul T, Greinacher A, Sachs UJ, et al. Inhibition of HPA-1a alloantibody-mediated platelet destruction by a deglycosylated anti-HPA-1a monoclonal antibody in mice: toward targeted treatment of fetal-alloimmune thrombocytopenia. Blood 2013;122:321-7. [Crossref] [PubMed]
- Bowman J. Thirty-five years of Rh prophylaxis. Transfusion 2003;43:1661-6. [Crossref] [PubMed]
- Kumpel BM. On the immunologic basis of Rh immune globulin (anti-D) prophylaxis. Transfusion 2006;46:1652-6. [Crossref] [PubMed]
- Tiller H, Killie MK, Chen P, et al. Toward a prophylaxis against fetal and neonatal alloimmune thrombocytopenia: induction of antibody-mediated immune suppression and prevention of severe clinical complications in a murine model. Transfusion 2012;52:1446-57. [Crossref] [PubMed]
- Geisen C, Fleck E, Schäfer SMG, et al. Rapid and complete clearance of HPA-1a mismatched platelets in a human model of fetal and neonatal alloimmune thrombocytopenia by a hyperimmune plasma derived polyclonal anti HPA-1a antibody. Res Pract Thromb Haemost 2021;5:abstr PB0969.
- Halle L, Bigot A, Mulen-Imandy G, et al. HPA polymorphism in sub-Saharan African populations: Beninese, Cameroonians, Congolese, and Pygmies. Tissue Antigens 2005;65:295-8. [Crossref] [PubMed]
- Van Der Weerdt CM, Veenhoven-Vonriesz LE, Nijenhuis LE, et al. The Zw Blood Group System in Platelets. Vox Sang 1963;8:513-30. [Crossref] [PubMed]
- Saji H, Maruya E, Fujii H, et al. New platelet antigen, Siba, involved in platelet transfusion refractoriness in a Japanese man. Vox Sang 1989;56:283-7. [Crossref] [PubMed]
- Grenet P, Dausset J, Dugas M, et al. Neonatal thrombopenic purpura with anti-Ko-a feto-maternal isoimmunization. Arch Fr Pediatr 1965;22:1165-74. [PubMed]
- Esquirol A, Canals C, Ibáñez M, et al. Foetal-neonatal alloimmune thrombocytopenia due to anti-HPA-2b antibodies present in the serum of a mother initially mistyped as HPA-1a negative. Blood Transfus 2012;10:390-2. [PubMed]
- Goldman M, Trudel E, Richard L, et al. Neonatal alloimmune thrombocytopenia due to anti-HPA-2b (anti-Koa). Immunohematology 2003;19:43-6. [Crossref] [PubMed]
- Bizzaro N, Dianese G. Neonatal alloimmune amegakaryocytosis. Case report. Vox Sang 1988;54:112-4. [PubMed]
- Kuijpers RW, Faber NM, Cuypers HT, et al. NH2-terminal globular domain of human platelet glycoprotein Ib alpha has a methionine 145/threonine145 amino acid polymorphism, which is associated with the HPA-2 (Ko) alloantigens. J Clin Invest 1992;89:381-4. [Crossref] [PubMed]
- Quach ME, Li R. Structure-function of platelet glycoprotein Ib-IX. J Thromb Haemost 2020;18:3131-41. [Crossref] [PubMed]
- Ulrichts H, Vanhoorelbeke K, Cauwenberghs S, et al. von Willebrand factor but not alpha-thrombin binding to platelet glycoprotein Ibalpha is influenced by the HPA-2 polymorphism. Arterioscler Thromb Vasc Biol 2003;23:1302-7. [Crossref] [PubMed]
- Clemetson KJ, Clemetson JM. Platelet GPIb-V-IX complex. Structure, function, physiology, and pathology. Semin Thromb Hemost 1995;21:130-6. [Crossref] [PubMed]
- Wu G, Essex DW, Meloni FJ, et al. Human endothelial cells in culture and in vivo express on their surface all four components of the glycoprotein Ib/IX/V complex. Blood 1997;90:2660-9. [Crossref] [PubMed]
- Eksteen M, Heide G, Tiller H, et al. Anti-human platelet antigen (HPA)-1a antibodies may affect trophoblast functions crucial for placental development: a laboratory study using an in vitro model. Reprod Biol Endocrinol 2017;15:28. [Crossref] [PubMed]
- Kelton JG, Smith JW, Horsewood P, et al. Gova/b alloantigen system on human platelets. Blood 1990;75:2172-6. [Crossref] [PubMed]
- Smith JW, Hayward CP, Horsewood P, et al. Characterization and localization of the Gova/b alloantigens to the glycosylphosphatidylinositol-anchored protein CDw109 on human platelets. Blood 1995;86:2807-14. [Crossref] [PubMed]
- Bordin JO, Kelton JG, Warner MN, et al. Maternal immunization to Gov system alloantigens on human platelets. Transfusion 1997;37:823-8. [Crossref] [PubMed]
- Moncharmont P, Courvoisier S, Pagnier A, et al. Severe HPA-15b related neonatal alloimmune thrombocytopenia. Acta Paediatr 2007;96:1701-3. [Crossref] [PubMed]
- Matsuhashi M, Tsuno NH, Sone S, et al. The role of alloantibodies against human platelet antigen-15 in multiply platelet transfused patients. Transfusion 2014;54:1093-9. [Crossref] [PubMed]
- Ertel K, Al-Tawil M, Santoso S, et al. Relevance of the HPA-15 (Gov) polymorphism on CD109 in alloimmune thrombocytopenic syndromes. Transfusion 2005;45:366-73. [Crossref] [PubMed]
- Feng ML, Liu DZ, Shen W, et al. Establishment of an HPA-1- to -16-typed platelet donor registry in China. Transfus Med 2006;16:369-74. [Crossref] [PubMed]
- Phuangtham R, Romphruk A, Puapairoj C, et al. Human platelet antigens in Burmese, Karen and north-eastern Thais. Transfus Med 2017;27:60-5. [Crossref] [PubMed]
- Berry JE, Murphy CM, Smith GA, et al. Detection of Gov system antibodies by MAIPA reveals an immunogenicity similar to the HPA-5 alloantigens. Br J Haematol 2000;110:735-42. [Crossref] [PubMed]
- Koh Y, Nishimiya H, Inoue H, et al. A novel simple assay system for the detection of human platelet antigen 15 (HPA-15) alloantibodies based on three techniques: an HPA-15 expressing cell line, a monoclonal antibody-specific antigen-capture method and mixed-passive haemagglutination. Vox Sang 2020;115:202-6. [Crossref] [PubMed]
- Brashem-Stein C, Nugent D, Bernstein ID. Characterization of an antigen expressed on activated human T cells and platelets. J Immunol 1988;140:2330-3. [PubMed]
- Haregewoin A, Solomon K, Hom RC, et al. Cellular expression of a GPI-linked T cell activation protein. Cell Immunol 1994;156:357-70. [Crossref] [PubMed]
- Murray LJ, Bruno E, Uchida N, et al. CD109 is expressed on a subpopulation of CD34+ cells enriched in hematopoietic stem and progenitor cells. Exp Hematol 1999;27:1282-94. [Crossref] [PubMed]
- Giesert C, Marxer A, Sutherland DR, et al. Antibody W7C5 defines a CD109 epitope expressed on CD34+ and CD34- hematopoietic and mesenchymal stem cell subsets. Ann N Y Acad Sci 2003;996:227-30. [Crossref] [PubMed]
- Hashimoto M, Ichihara M, Watanabe T, et al. Expression of CD109 in human cancer. Oncogene 2004;23:3716-20. [Crossref] [PubMed]
- Zhang JM, Hashimoto M, Kawai K, et al. CD109 expression in squamous cell carcinoma of the uterine cervix. Pathol Int 2005;55:165-9. [Crossref] [PubMed]
- Cuppini L, Calleri A, Bruzzone MG, et al. Prognostic value of CD109+ circulating endothelial cells in recurrent glioblastomas treated with bevacizumab and irinotecan. PLoS One 2013;8:e74345. [Crossref] [PubMed]
- Prosper JYA. Characterization of CD109. PhD Thesis. Department of Medical Biophysiscs. University of Toronto. Available online: https://tspace.library.utoronto.ca/bitstream/1807/29842/1/Prosper_Joseph_Y_201106_PhD_thesis.pdf
- Maślanka K, Michur H, Guz K, et al. The relevance of HPA-15 antigen expression for anti-HPA-15 antibody detection. Int J Lab Hematol 2012;34:65-9. [Crossref] [PubMed]
- Suciu-Foca N, Reed E, Rubinstein P, et al. A late-differentiation antigen associated with the helper inducer function of human T cells. Nature 1985;318:465-7. [Crossref] [PubMed]
- Ye BG, Sun HC, Zhu XD, et al. Reduced expression of CD109 in tumor-associated endothelial cells promotes tumor progression by paracrine interleukin-8 in hepatocellular carcinoma. Oncotarget 2016;7:29333-45. [Crossref] [PubMed]
Cite this article as: Xu X, Fu Y, Kiefel V, Santoso S. Fetal and neonatal immune thrombocytopenia caused by maternal alloantibodies and isoantibodies in Caucasian and Asian populations: a narrative review. Ann Blood 2021;6:36.