FcRn antagonists in ITP
Introduction
ITP is an acquired autoimmune bleeding disorder characterized by a low platelet count (<100×109/L) in the absence of other causes or disorders associated with thrombocytopenia (1-3). Depending on the level of reduction in the platelet count there is an increased risk of bleeding, ranging from skin and mucosal bleeding, menorrhagia, gastrointestinal bleeding and in the most severe intracranial hemorrhage (1,4,5). It has also been recognised that there is an increased incidence of depression and fatigue (6) as well as side effects of existing therapies, which all have an impact on quality of life (7-12). There are a number of different therapeutic approaches and, traditionally non-specific immunosuppression (e.g., steroids, rituximab and other immune suppressive therapies), and inhibition of platelet clearance [e.g., splenectomy, intravenous immunoglobulin (IVIg), anti-D immunoglobulin, and the recently FDA-approved Syk inhibitor fostamatinib (13)] have been the mainstay of treatment. Over the last decade stimulation of platelet production [e.g., thrombopoietin receptor agonists (TPO-RA)] (4,14) has come increasingly to the fore and has been an important addition to the treatment options. Splenectomy remains the only treatment that provides sustained remission off therapy for a high proportion of patients (2,3) but has increasingly fallen out of favour as newer splenectomy sparing options have become available.
Although the pathogenesis of ITP is complex in nearly two-thirds of patient autoantibodies, predominantly of the IgG class can be detected. These mediate their pathogenic actions by targeting surface glycoproteins (GP) which are expressed on both platelets and megakaryocytes, the progenitor cells of platelets (15,16). Detected in approximately 60% of patients with ITP (17), they contribute to the platelet degradation, induction of platelet apoptosis, impairment of platelet production and platelet function (18-25). The importance of IgG autoantibodies in the development of ITP is supported by the observation of a low platelet count in infants born to mothers with ITP, due to placental transfer of autoantibodies (26,27). Older IgG-depleting treatment modalities, such as immunoadsorption and plasmapheresis, which lead to a reduction of platelet-associated autoantibodies (26) and an associated platelet count (28) increment support the central importance of autoantibodies.
The neonatal Fc Receptor (FcRn) is acknowledged as the central regulator of IgG homeostasis, protecting the IgG from lysosomal degradation and as a consequence increasing their half-life (29,30). FcRn also recycles albumin but binds at a site distinct from that of IgGs (31). The identification of FcRn as a integral component in the regulation of IgG homeostasis stimulated the development of FcRn antagonists. In healthy volunteers, FcRn antagonists, such as efgartigimod, a human IgG1 antibody Fc-fragment, or rozanolixizumab, an IgG4 monoclonal antibody (mAb), had no significant adverse events and were well tolerated. They induced a rapid reduction in total IgGs involving all IgG subtypes (32,33). The concept of targeted reduction of IgG autoantibodies through FcRn blockade to prevent their pathogenic actions has then been demonstrated in patients with ITP in Phase 2 clinical trials and is currently being evaluated in Phase 3 clinical trials.
In this review article, we discuss the role of FcRn as the central regulator of IgG homeostasis, the therapeutic approaches involving IgG reduction as well as the clinical trial results of two FcRn antagonists currently being investigated in Phase 3 clinical trials in patients with ITP, efgartigimod and rozanolixizumab.
FcRn is the central regulator of IgG homeostasis
FcRn roles
FcRn is a major histocompatibility complex (MHC) class I-related receptor, interacting with antibodies of the IgG class as a heterodimer of a heavy α-chain non-covalently associated with β2-microglobulin, which binds to the constant or fragment crystallizable (Fc) region of the IgG subclasses (Figure 1) (34). Given that IgG is a homodimeric molecule and contains two Fc domains, FcRn-IgG interactions have been proposed to occur with a stoichiometry of two FcRn molecules per one IgG (2:1) (35), FcRn is a key player in regulating the dynamic behavior, including distribution, transport and persistence of IgG antibodies throughout the body, playing an important role in IgG homeostasis throughout life (36,37), FcRn may also have a role on antigen presentation, which could mean that its long-term blockade could affect autoimmune responses since the plasma cells producing the IgG autoantibodies would be deprived from survival signals linked to antigen presentation (38), The FcRn-mediated IgG recycling rate is estimated to be 42% greater than the IgG production rate, indicating that the recycling of IgG, not its production, is the dominant process for maintaining the IgG serum concentration in human (39), The serum concentration of IgGs is therefore controlled not only by antigen-driven production of IgGs, but by FcRn-mediated salvage of IgGs from degradation (39), The discovery of these activities extends the role of this Fc receptor well beyond its original identification as the transporter of IgGs from mother to developing fetus (hence the name n, for neonatal). Indeed, FcRn was originally isolated from the rat intestinal epithelial cell brush borders (40-43), During gestation in humans, FcRn mediates the transfer of IgGs from mother to fetus across the placenta, providing newborns with humoral immunity (35,44), FcRn is widely distributed in various mammalian organs, tissues and cells, it is most highly expressed in hematopoietic cells, intestinal epithelia, and in the vascular endothelium, particularly in skin and skeletal muscle (45-47),
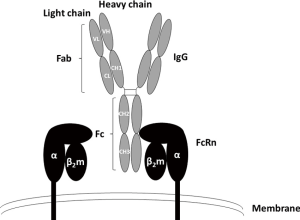
FcRn as a regulator of the half-live of IgGs
FcRn binds to the Fc region of IgGs at a distinct site from the binding sites of the classical Fc receptors for IgGs (FcγRs) or the C1q component of complement which binds near the upper CH2 domain and hinge region, which initiates the classical pathway of complement activation (Figure 1) (48,49), The IgG-FcRn interface is composed of three subsites, a hydrophobic core and two electrostatic sites. IgG residues that are critical for the binding of human IgGs to FcRn include isoleucine (Ile)253, histidine (His)310, His435, and tyrosine (Tyr)436. These are located on the exposed loops at the CH2-CH3 domain interface (30,49-51). In general, these amino acids show higher variability, such as residues 435 and 436, than Ile253 and His310 across different IgG isotypes (52). The His residues of IgG interact with acidic residues of FcRn (49,53). The interaction of the protonated imidazole side chain of His (pKa ~6) with these acidic residues at pH ~6 confers the characteristic pH dependent binding (relatively high affinity at acidic pH, with very weak to negligible binding at pH 7.3–7.4) that is observed for the majority of IgGs (54,55). Therefore, FcRn binds to the Fc region of IgG in a strictly pH-dependent manner in contrast to FcγRs and other Fc-binding proteins; at physiological pH 7.4, FcRn does not bind IgGs, but has a low micromolar to nanomolar affinity for the Fc region of IgGs at the acidic pH of the endosome (pH 6–6.5) (29). FcRn-IgG complexes are then internalized primarily by fluid phase pinocytosis (due to the very low affinity of FcRn for most IgG subclasses at extracellular, near neutral pH), sorted in early (sorting) endosomes away from lysosomal degradation and recycled back to the cell surface for exocytic release (Figure 2) (56-58). IgGs that do not bind FcRn, possibly due to saturation of FcRn interaction sites or IgG mutations that ablate FcRn binding, proceed through lysosomal degradation (59,60). FcRn recycling of IgGs results in the higher concentration and longer half-life of IgGs (T1/2 ≈21 days) compared with other immunoglobulins, such as IgA (T1/2 ≈5.8 days) and IgM (T1/2 ≈5.1 days), which are not recycled by FcRn (42,61-63). As has been previously mentioned albumin is also recycled by FcRn, but it is known to bind at a site distinct from that of IgGs (64). This interaction is also pH-dependent (65).
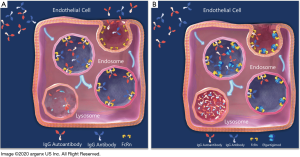
Pathogenic properties of IgG autoantibodies in autoimmune diseases
Elevated levels of IgG autoantibodies are associated with many autoimmune diseases, including myasthenia gravis (MG), systemic lupus erythematosus, rheumatoid arthritis, chronic inflammatory demyelinating polyradiculoneuropathy (CIDP), pemphigus vulgaris (PV), and ITP (34,66-71). The position of FcRn as central regulator of IgG serum levels led to study of its manipulation in controlling the levels of IgG autoantibodies. While the mechanism by which IgG autoantibodies are initiated in ITP (72) is not entirely clear, they are central to the clinical impact of the disease by targeting surface GPs expressed on platelets and megakaryocytes (15-17). The IgG1 subclass of IgG immunoglobulin are the predominant form identified, while IgG2, IgG3, and IgG4 subclass autoantibodies may be present they are usually in association with IgG1 antibodies (73,74). Detected in approximately 60% of patients with ITP (17), IgG autoantibodies will, in general, opsonize platelets, resulting in clearance by splenic macrophages, inducing platelet apoptosis (18), complement-dependent lysis (19) or desialylation of platelets leading to Fc-independent liver clearance (Figure 3A,B) (20). A more recent finding is their ability to inhibit megakaryocyte proliferation and differentiation resulting in reduced platelet production (Figure 3C) (21-23). Some of the anti-GP antibodies also interfere with platelet function, leading to an inhibition of platelet aggregation (24) and therefore blood clot formation (Figure 3D) (25). The majority of anti-GP autoantibodies is directed against GPIIb/IIIa (~70%) and/or GPIb-IX-V complex (~25%) (75,76), but antibodies against GPIa-IIa or GPVI are also detected in sporadic cases (~5%) (17),
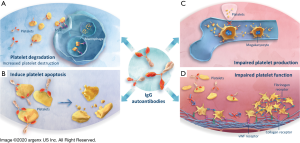
Established therapies reducing IgG autoantibodies
Therapeutic plasma exchange
Plasma exchange is a procedure that separates and removes the patient’s plasma allowing to get rid of the IgG autoantibodies. The plasma that is removed from the patient must be replaced to maintain volume and replenish other essential components. Commonly replacement fluids will contain some proportion of another protein solution, such as 5% human albumin. The standard dose is one plasma volume exchange once per day for one to eight days (77). Reduction in IgG levels correlates with improvement in ITP manifestations (29–80% response rate) (77), providing proof-of-concept that reduction of IgG levels through FcRn antagonism is a viable therapeutic option. Plasma exchange is frequently associated with a mix of side effects including fatigue, nausea, dizziness, feeling cold and tingling in the fingers and around the mouth. Allergic reaction and lowered blood pressure can be a particular problem (28,78,79).
Protein A immunoadsorption
Evidence of efficacy has been shown for extracorporeal immunoadsorption of plasma as a therapy for adults with treatment-resistant ITP to remove IgGs and circulating immune complexes. An average of six treatments (0.25 to 2.0 L plasma per treatment) is generally given over two to three weeks (77). Clinical responses were associated with 21% response rate and significant decreases in specific serum platelet autoantibodies (including anti-GP IIb/IIIa), platelet-associated immunoglobulin, and circulating immune complexes (77). However, hypersensitivity reactions, pain, nausea/vomiting, and cardiopulmonary complications have been associated to this therapy (26,27).
High-dose intravenous immunoglobulin
IVIg products are prepared from the pooled plasma of healthy donors. It consists of over 95% IgGs with a subclass distribution corresponding to that in normal human serum (80). The use of high-dose IVIg for the treatment of ITP in both children and adults was shown more than four decades ago (81,82). The dose regimens used are variable but were initially given as 0.4 g/kg daily for 5 days. More recently it has been accepted that 1 g/kg IVIg is effective in the majority of recipients with two-thirds normalising their platelet count with fewer than 10% failing to elicit any response. This is seen even those not responding to corticosteroids (83). Overall, response rates are similar to those of corticosteroids, but with a shorter time to response. This may last up to a week but is frequently as short as 24–48 hours (84). IVIg treatment has a significant cost and is associated with frequent side effects, the most common being headache, which may occur in up to 30%, and skin rashes. More rarely but of greater severity are renal failure and thrombosis although these are less common with the newer formulations, which have low sucrose content (83). Due to the cost and short response duration, IVIg is considered more as a rescue option in ITP than routine first-line treatment and is often used as a bridge until other treatments have had an effect (2,3). Therefore its use is reserved for patients with severe bleeding as part of combination therapy, as preparation for an urgent invasive procedure or as bridging to more definitive second-line treatment in a unresponsive patient population. There are several theories as to the mechanism of IVIg action and it is likely that it acts multiple mechanisms. The most likely early mechanism of response is by inhibition of FcγR-mediated platelet destruction but it is also known to have immunomodulatory effects including anti-idiotypic neutralization of antiplatelet antibodies and stimulation of FcγRIIB expression (85). There is also a suggestion that it may also work through competitive inhibition of FcRn and IVIg-induced acceleration of antiplatelet antibody elimination (86).
IgG autoantibodies reduction through FcRn blocking
As indicated previously, FcRn is the predominant regulator of IgG levels, recycling IgGs at a faster rate than IgG production (39). Given the pathogenic roles of IgG autoantibodies in ITP and the proven efficacy of existing treatments capable of reducing IgG levels (e.g., therapeutic plasma exchange, protein A immunoadsorption, and IVIg), blocking the recycling of IgGs by FcRn represents a rational and promising approach for the treatment of patients with ITP. An understanding of the biochemistry of FcRn and on its interaction with IgGs stimulated further study, which was aided by the generation of FcRn knock-out and human FcRn transgenic mice. Recombinant soluble human FcRn can be produced in bacteria in high amounts, with the recombinant receptor reproducing the characteristic pH-dependent reversible binding to IgGs at pH 6.0, with almost undetectable binding at neutral pH (87). However, experimental evidence demonstrating its transfer into intracellular acidic compartments of endosomes where IgG binding to FcRn should occur, is lacking, indicating that it could not be used as a therapeutic approach. It was therefore considered that inhibition of the IgG-FcRn interaction was probably best achieved by using FcRn-specific blocking agents (Figure 2B) (88). There are a range of FcRn inhibitors from peptide/small protein to antibody-based blockers which have been generated, and many are in various stages of clinical development following preclinical analyses in animal models (Table 1).
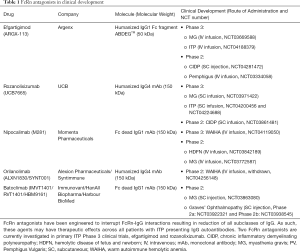
Full table
FcRn antagonists in clinical development
Antibody fragments directed against FcRn
One class of FcRn antagonists involves the use of Fc engineering to generate competitive IgG molecules with substantially increased affinity for FcRn at both near neutral and acidic pH (89), such as efgartigimod, a human IgG1 antibody Fc-fragment (Table 1) (32). Unlike mAbs, efgartigimod does not have a Fab region. Instead, efgartigimod binds to FcRn via its Fc region, which is the same way that endogenous IgGs binds to FcRn (34,90). This molecule is a natural ligand of FcRn that has been engineered with ABDEGTM (for AntiBodies that enhance IgG DEGradation) mutations [methionine (Met)252 to Tyr, serine (Ser)254 to threonine (Thr), Thr256 to glutamic acid (Glu), His433 to lysine (Lys), and asparagine (Asn)434 to phenylalanine (Phe)], which are located in the CH2 and CH3 domain of the Fc region. The impact of these mutations is to increase affinity for FcRn. Due to its superior 100-fold increased affinity for FcRn at pH 6.0 (KD efgartigimod =15.5 nM vs. KD wild-type human IgG1 =370 nM) and the retained significant binding activity at pH 7.2 (vs. no binding detected for wild-type human IgG1), efgartigimod therefore outcompetes IgGs for binding to FcRn (89). The impact of this results in accelerated degradation of endogenous IgGs (Figure 2B) (29,89,91). By contrast, mAbs that bind through their variable domains typically involve distinct sets of interacting residues and do not have this property (90). Due to the pH-dependent binding and especially the relative poor affinity at neutral pH, efgartigimod is able to dissociate from the complex with FcRn leading to a serum half-life that has been reported to be longer than for the FcRn targeting mAbs (i.e., approximately 85.1 to 104 hours over 2.0 to 50 mg/kg) (32).
Monoclonal antibodies directed against FcRn
The other class of FcRn antagonists consists of fully human and humanized mAbs, which have been engineered such that their Fab portions binds FcRn with high affinity and prevent the binding of wild type IgG Fc regions with FcRn. These mAbs ultimately outcompete binding of IgG autoantibodies and force their degradation, such as rozanolixizumab (Table 1).
Clinical Trial Results of Efgartigimod, a Novel FcRn Antibody Fragment Antagonist
Phase 1 study
A first-in-human, randomized, double-blind, placebo-controlled, dose-escalating study was conducted in 62 healthy volunteers to explore single and multiple ascending intravenous (IV) doses of efgartigimod (NCT03457649) (32). The study objectives were to assess safety, tolerability, pharmacokinetics (PK), pharmacodynamics (PD), and immunogenicity. Importantly efgartigimod did not alter the homeostasis of either albumin or Igs other than IgGs, and no serious adverse events related to efgartigimod infusion were observed. One serious adverse event of hyperventilation was reported but was considered unlikely to be related to efgartigimod treatment. Single administration of efgartigimod (0.2, 2, 10, 25 or 50 mg/kg) reduced baseline IgG levels by approximately 50%, while multiple dosing (10 mg/kg q4d, 10 mg/kg q7d or 25 mg/kg q7d) further lowered IgG levels by an average of 75% from baseline levels, with 10 mg/kg q7d chosen as the ideal dose. IgG levels were observed to return to baseline approximately eight weeks after cessation of administration. This sustained PD effect contrasts with the PK profile of efgartigimod, which demonstrated a rapid reduction in serum concentrations of the compound following the last dosing. These findings suggest that most of the administered efgartigimod is rapidly bound to FcRn, antagonizing its function for a prolonged period.
Phase 2 study
In a randomized, double-blind, placebo-controlled Phase 2 study (NCT03102593), 38 patients were randomized 1:1:1 to receive 4 weekly intravenous infusions of either placebo (N=12) or efgartigimod at a dose of 5 mg/kg (N=13) or 10 mg/kg (N=13) (92). Patients who were predominantly refractory, or who had relapsed following previous lines of therapy were included in the study. This short treatment cycle of efgartigimod in patients with ITP, was designed to look at tolerability and to confirm the favourable safety profile seen in the Phase 1 studies. Following infusion of efgartigimod there was a rapid reduction of total IgG levels (up to 63.7% mean change from baseline), which was associated with clinically relevant increases in platelet counts (46% patients on efgartigimod vs. 25% on placebo achieved a platelet count of ≥50×109/L on at least two occasions and 38% vs. 0% achieved ≥50×109/L for at least 10 cumulative days). Although patients with severe bleeding were not entered into the randomised study there was also a reduced proportion of patients with minor bleeding episodes. There was also a parallel reduction in platelet-associated autoantibody (GPIIb/IIIa, GPIb/IX, and GPIa/IIa) levels.
Phase 3 study
A Phase 3, multicenter, randomized, double-blinded, placebo-controlled trial (NCT04188379), evaluates the efficacy and safety of efgartigimod in adults with persistent or chronic primary ITP. Trial participants will be eligible for continuation into a long-term open-label extension trial (NCT04225156).
Other indications
After positive results obtained in a Phase 2 proof-of-concept trial investigating the use of efgartigimod in patients with MG (NCT02965573) (93), positive topline Phase 3 results have been announced (NCT03669588). Phase 2 clinical trials investigating the use of efgartigimod in patients with CIDP (NCT04281472) and pemphigus (NCT03334058) are ongoing.
Clinical trial results of rozanolixizumab, a novel FcRn antagonist
Phase 1 study
A first-in-human, randomized, double-blind, placebo-controlled, dose-escalating study of IV or subcutaneous (SC) rozanolixizumab was conducted in healthy subjects (NCT02220153). The primary objective was to evaluate safety and tolerability with the secondary objectives of assessing the PK and PD, and the impact on IgG levels. Forty-nine subjects were randomized into 6 cohorts to receive rozanolixizumab (n=36) or placebo (n=13). Rozanolixizumab was given to 3 cohorts as an IV preparation, and the final 3 subcutaneously. The SC doses given were 1, 4, or 7 mg/kg (n=6 for each cohort; plus n=7 or 6 for placebo, respectively). The most frequent treatment-emergent adverse event [TEAE]; headache, 14 of 36 (38.9%) subjects]. This was dose-dependent and was more prominent after IV administration. Severe TEAEs occurred in four subjects, all in the highest-dose IV group [headache (n=3) and back pain (n=1)]. The safety profile of IV doses of 7 mg/kg precluded the use of higher doses in this study of healthy individuals. There were sustained dose-dependent reductions in serum IgG concentrations (IV and SC rozanolixizumab). IgG concentrations in the serum were reduced by up to 50% in this study, which is similar to the 50% to 60% reductions observed after plasma exchange (94,95). Rozanolixizumab PK demonstrated nonlinear increases with dose.
Phase 2 study
The Phase 2 study to assess the safety, tolerability and efficacy of rozanolixizumab was designed to explore a multiple dose regimen in order to inform the dosing strategy for further development in ITP (NCT02718716). Sixty-six patients received either a single dose (1×15 mg/kg or 1×20 mg/kg) or multiple doses (5×4 mg/kg, 3×7 mg/kg, 2×10 mg/kg weekly) of SC rozanolixizumab. The total weekly dose was similar in all treatment groups, ranging from 15 to 21 mg/kg. The target platelet count was ≥50×109/L) and was seen across all groups in > 50% of patients and this coincided with the lowest mean IgG levels. There was a higher response rate (55-67% in 1×15 mg/kg and 1×20 mg/kg dose groups vs. 36–45% in 5×4, 3×7 and 2×10 mg/kg dose groups) and shorter time to response achieved by the 1×15 and 1×20 mg/kg rozanolixizumab dose groups (96). Results confirm that rozanolixizumab was well tolerated across all dose groups (96), consistent with previous rozanolixizumab studies. There is no specific description of the effect on autoantibody levels but the assumption is that that these paralleled the fall in IgG. The most commonly reported adverse event was headache, with mild-to-moderate headaches seen at higher doses; other reported adverse events included diarrhea and vomiting. These events were usually of short duration and the majority of events resolved without treatment. No patient discontinued the study due to side effects (96).
Phase 3 study and other indications
Phase 3 clinical trials investigating the use of rozanolixizumab in patients with primary ITP (NCT04200456 and NCT04224688) and MG (NCT03971422) are ongoing, as well as a Phase 2 in patients with CIDP (NCT03861481).
Comparison of different FcRn antagonists
The clinical trials results described above have shown that FcRn antagonists effectively reduce IgG levels with no impacts on levels of other immunoglobulins (32). This is in contrast to other common ITP treatments, such as steroids and non-steroidal immunosuppressants, which cause a wide-ranging depression of the immune system (97), and therapeutic plasma exchange, which removes normal and pathogenic IgGs, but depletes the blood of other Ig isotypes (such as IgM and IgA), coagulation factors, complement proteins, and albumin-bound medications (98).
Reduction in albumin levels were reported for nipocalimab (99), rozanolixizumab (33), and batoclimab (100) whereas this finding was not observed for the efgartigimod and orilanolimab (Table 1). Albumin is also recycled via FcRn, but binds to a different epitope, suggesting that IgG and albumin can bind simultaneously to FcRn (101). The full-length mAbs have picomolar affinities, with no difference between pH 6.0 and pH 7.4 in contrast to efgartigimod, for example, which has a pH-dependent affinity in the nanomolar range (89). Interestingly, previous studies have shown that when there is crosslinking of FcRn by multivalent immune complexes, such as full-length mAbs, there is an increase in lysosomal trafficking of FcRn-ligand complexes and immune activation (102,103).
To date, the various FcRn antagonists in clinical development display a favourable safety profile with the majority of TEAEs being mild. Interestingly, SC administration of rozanolixizumab was better tolerated than IV administration in healthy volunteers with occurrence of dose-dependent headaches and back pain, including four severe TEAEs in the latter group (33). Dose-dependent vomiting, nausea, and pyrexia were also seen more frequently with the IV formulation compared to placebo and were less frequent with the SC formulation. As a result of these findings, subsequent clinical development was continued using SC administration only to avoid high Cmax levels of the mAb. The improved tolerability profile of the SC administration was confirmed in patients with ITP, but when higher FcRn saturating doses were used the headaches were observed again (33,96). A similar TEAE profile was reported for orilanolimab (104), which is a humanized IgG4 like rozanolixizumab. Interestingly, neither nipocalimab (99) nor batoclimab (105), both humanized IgG1 mAbs (Fc engineered to take away effector functions), have reported this issue. These side effects may therefore be caused by FcγR-mediated effects. IgG4 antibodies differ functionally from the other IgG subclasses in their anti-inflammatory activity, making them the preferred subclass for applications where recruitment of immune effector functions is unnecessary. Nevertheless, it has been shown that the CD28 agonistic IgG4 mAb, TGN1412, via residual interactions with high affinity FcγR induced T-cell proliferation leading to the cytokine storm (106). Efgartigimod due to its ABDEGTM mutations has diminished affinity for FcγRs (107), which may explain that headaches and gastrointestinal problems were not observed with this drug.
Conclusions
Primary ITP is an acquired autoimmune bleeding disorder, characterized by a low platelet count (<100×109/L). In up to two-thirds of patients, IgG autoantibodies directed against platelet receptors can be detected. Their primary impact is to enhance platelet clearance and destruction. They are also known to have a significant inhibitory effect on platelet production, and may also impair platelet function. The overall impact is that as the platelet count falls there is an increased incidence of spontaneous bruising and an increased risk of excessive, and occasionally life-threatening, bleeding. More recently it has been recognized that there is also an associated increase in fatigue and tiredness with an impact on quality of life. FcRn is the central regulator of IgG homeostasis, rescuing IgGs from lysosomal degradation, prolonging their half-life, and promoting tissue distribution of IgGs. While FcRn-mediated half-life extension is beneficial for IgG responses against pathogens, it also prolongs the serum half-life of IgG autoantibodies promoting therefore autoimmune diseases and their chronicity. Drugs that block FcRn-mediated recycling of IgGs could reduce all IgG subtypes without impacting concentrations of other immunoglobulin isotypes, allowing these therapies to potentially treat a broad patient population with reduced side effects (Figure 4). This form of targeted therapy by specifically reducing only IgG has a potential advantage over broader immunosuppressive treatments which may lead to bone marrow suppression, in addition to a more non-specific immune suppression with a more general infection risk.
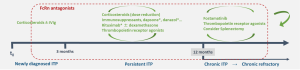
One antibody fragment and four fully human/humanized mAbs are currently in various phases of clinical development. Collectively, the clinical data obtained from these FcRn antagonists suggest that targeted IgG reduction is a potential new treatment modality in primary ITP, and warrants further evaluation of longer-term treatment in larger Phase 3 studies to fully demonstrate the potential of this strategy by providing a safe and effective treatment for patients with ITP.
Acknowledgments
Funding: None.
Footnote
Provenance and Peer Review: This article was commissioned by the Guest Editors (John W. Semple and Rick Kapur) for the series “Treatment of Immune Thrombocytopenia (ITP)” published in Annals of Blood. The article was sent for external peer review organized by the Guest Editors and the editorial office.
Conflicts of Interest: Both authors have completed the ICMJE uniform disclosure form (available at http://dx.doi.org/10.21037/aob-20-55). The series “Treatment of Immune Thrombocytopenia (ITP)” was commissioned by the editorial office without any funding or sponsorship. Adrian C. Newland is a consultant for Amgen, Angle, argenx, Dova, Novartis, Ono, Rigel, and Shionogi; received funding from Amgen, Novartis, and Rigel; received honoraria directly from Amgen, Angle, argenx, Dova, Novartis, Ono, Rigel, and Shionogi; and paid expert testimony from argenx and Rigel. Vickie McDonald has received research support from Baxter and Grifols and advisory fees for Alexion. The authors have no other relevant affiliations or financial involvement with any organization or entity with a financial interest in or financial conflict with the subject matter or materials discussed in the manuscript apart from those disclosed.
Ethical Statement: The authors are accountable for all aspects of the work in ensuring that questions related to the accuracy or integrity of any part of the work are appropriately investigated and resolved.
Open Access Statement: This is an Open Access article distributed in accordance with the Creative Commons Attribution-NonCommercial-NoDerivs 4.0 International License (CC BY-NC-ND 4.0), which permits the non-commercial replication and distribution of the article with the strict proviso that no changes or edits are made and the original work is properly cited (including links to both the formal publication through the relevant DOI and the license). See: https://creativecommons.org/licenses/by-nc-nd/4.0/.
References
- Kistangari G, McCrae KR. Immune thrombocytopenia. Hematol Oncol Clin North Am 2013;27:495-520. [Crossref] [PubMed]
- Neunert C, Terrell DR, Arnold DM, et al. American Society of Hematology 2019 guidelines for immune thrombocytopenia. Blood Adv 2019;3:3829-66. [Crossref] [PubMed]
- Provan D, Arnold DM, Bussel JB, et al. Updated international consensus report on the investigation and management of primary immune thrombocytopenia. Blood Adv 2019;3:3780-817. [Crossref] [PubMed]
- Zufferey A, Kapur R, Semple J. Pathogenesis and Therapeutic Mechanisms in Immune Thrombocytopenia (ITP). J Clin Med 2017;6:16. [Crossref] [PubMed]
- Cohen YC, Djulbegovic B, Shamai-Lubovitz O, et al. The bleeding risk and natural history of idiopathic thrombocytopenic purpura in patients with persistent low platelet counts. Arch Intern Med 2000;160:1630-8. [Crossref] [PubMed]
- Hill QA, Newland AC. Fatigue in immune thrombocytopenia. Br J Haematol 2015;170:141-9. [Crossref] [PubMed]
- McMillan R, Bussel JB, George JN, et al. Self-reported health-related quality of life in adults with chronic immune thrombocytopenic purpura. Am J Hematol 2008;83:150-4. [Crossref] [PubMed]
- Snyder CF, Mathias SD, Cella D, et al. Health-related quality of life of immune thrombocytopenic purpura patients: results from a web-based survey. Curr Med Res Opin 2008;24:2767-76. [Crossref] [PubMed]
- Doobaree IU, Nandigam R, Bennett D, et al. Thromboembolism in adults with primary immune thrombocytopenia: a systematic literature review and meta-analysis. Eur J Haematol 2016;97:321-30. [Crossref] [PubMed]
- Sarpatwari A, Bennett D, Logie JW, et al. Thromboembolic events among adult patients with primary immune thrombocytopenia in the United Kingdom General Practice Research Database. Haematologica 2010;95:1167-75. [Crossref] [PubMed]
- Sarpatwari A, Watson S, Erqou S, et al. Health-related lifestyle in adults and children with primary immune thrombocytopenia (ITP). Br J Haematol 2010;151:189-91. [Crossref] [PubMed]
- Mathias SD, Gao SK, Miller KL, et al. Impact of chronic Immune Thrombocytopenic Purpura (ITP) on health-related quality of life: a conceptual model starting with the patient perspective. Health Qual Life Outcomes 2008;6:13. [Crossref] [PubMed]
- Bussel J, Arnold DM, Grossbard E, et al. Fostamatinib for the treatment of adult persistent and chronic immune thrombocytopenia: Results of two phase 3, randomized, placebo-controlled trials. Am J Hematol 2018;93:921-30. [Crossref] [PubMed]
- Cines DB, Blanchette VS. Immune thrombocytopenic purpura. N Engl J Med 2002;346:995-1008. [Crossref] [PubMed]
- Nugent D, McMillan R, Nichol JL, et al. Pathogenesis of chronic immune thrombocytopenia: increased platelet destruction and/or decreased platelet production. Br J Haematol 2009;146:585-96. [Crossref] [PubMed]
- Stasi R. Immune thrombocytopenia: pathophysiologic and clinical update. Semin Thromb Hemost 2012;38:454-62. [Crossref] [PubMed]
- Swinkels M, Rijkers M, Voorberg J, et al. Emerging concepts in immune thrombocytopenia. Front Immunol 2018;9:880. [PubMed]
- Goette NP, Glembotsky AC, Lev PR, et al. Platelet apoptosis in adult immune thrombocytopenia: insights into the mechanism of damage triggered by auto-antibodies. PLoS One 2016;11:e0160563. [Crossref] [PubMed]
- Najaoui A, Bakchoul T, Stoy J, et al. Autoantibody‐mediated complement activation on platelets is a common finding in patients with immune thrombocytopenic purpura (ITP). Eur J Haematol 2012;88:167-74. [Crossref] [PubMed]
- Li J, van der Wal DE, Zhu G, et al. Desialylation is a mechanism of Fc-independent platelet clearance and a therapeutic target in immune thrombocytopenia. Nat Commun 2015;6:7737. [Crossref] [PubMed]
- Houwerzijl EJ, Blom NR, van der Want JJ, et al. Ultrastructural study shows morphologic features of apoptosis and para-apoptosis in megakaryocytes from patients with idiopathic thrombocytopenic purpura. Blood 2004;103:500-6. [Crossref] [PubMed]
- Chang M, Nakagawa PA, Williams SA, et al. Immune thrombocytopenic purpura (ITP) plasma and purified ITP monoclonal autoantibodies inhibit megakaryocytopoiesis in vitro. Blood 2003;102:887-95. [Crossref] [PubMed]
- McMillan R, Wang L, Tomer A, et al. Suppression of in vitro megakaryocyte production by antiplatelet autoantibodies from adult patients with chronic ITP. Blood 2004;103:1364-9. [Crossref] [PubMed]
- Al-Samkari H, Van Cott EM, Kuter DJ. Platelet aggregation response in immune thrombocytopenia patients treated with romiplostim. Ann Hematol 2019;98:581-8. [Crossref] [PubMed]
- Middelburg RA, Carbaat-Ham JC, Hesam H, et al. Platelet function in adult ITP patients can be either increased or decreased, compared to healthy controls, and is associated with bleeding risk. Hematology 2016;21:549-51. [Crossref] [PubMed]
- Snyder HW, Cochran SK, Balint J, et al. Experience with protein A-immunoadsorption in treatment-resistant adult immune thrombocytopenic purpura. Blood 1992;79:2237-45. [Crossref] [PubMed]
- Cahill MR, Macey M, Cavenagh J, et al. Protein A immunoadsorption in chronic refractory ITP reverses increased platelet activation but fails to achieve sustained clinical benefit. Br J Haematol 1998;100:358-64. [Crossref] [PubMed]
- Blanchette VS, Hogan V, McCombie N, et al. Intensive plasma exchange therapy in ten patients with idiopathic thrombocytopenic purpura. Transfusion 1984;24:388-94. [Crossref] [PubMed]
- Roopenian DC, Akilesh S. FcRn: the neonatal Fc receptor comes of age. Nat Rev Immunol 2007;7:715-25. [Crossref] [PubMed]
- Challa DK, Velmurugan R, Ober RJ, et al. FcRn: from molecular interactions to regulation of IgG pharmacokinetics and functions. Curr Top Microbiol Immunol 2014;382:249-72. [Crossref] [PubMed]
- Sand KMK, Bern M, Nilsen J, et al. Unraveling the interaction between FcRn and albumin: opportunities for design of albumin-based therapeutics. Front Immunol 2015;5:682. [Crossref] [PubMed]
- Ulrichts P, Cousin T, Dreier T, et al. Argx-113, a novel Fc-based approach for antibody-induced pathologies such as primary immune thrombocytopenia. American Society of Hematology Washington, DC; 2016.
- Kiessling P, Lledo-Garcia R, Watanabe S, et al. The FcRn inhibitor rozanolixizumab reduces human serum IgG concentration: A randomized phase 1 study. Sci Translat Med 2017;9:eaan1208.
- Ward ES, Ober RJ. Targeting FcRn to generate antibody-based therapeutics. Trends Pharmacol Sci 2018;39:892-904. [Crossref] [PubMed]
- Pyzik M, Sand KM, Hubbard JJ, et al. The neonatal Fc receptor (FcRn): A misnomer? Front Immunol 2019;10:1540. [Crossref] [PubMed]
- Huber AH, Kelley RF, Gastinel LN, et al. Crystallization and stoichiometry of binding of a complex between a rat intestinal Fc receptor and Fc. J Mol Biol 1993;230:1077-83. [Crossref] [PubMed]
- Raghavan M, Gastinel LN, Bjorkman PJ. The class I major histocompatibility complex related Fc receptor shows pH-dependent stability differences correlating with immunoglobulin binding and release. Biochemistry 1993;32:8654-60. [Crossref] [PubMed]
- Rath T, Baker K, Pyzik M, et al. Regulation of immune responses by the neonatal fc receptor and its therapeutic implications. Front Immunol 2015;5:664. [Crossref] [PubMed]
- Kim J, Hayton WL, Robinson JM, et al. Kinetics of FcRn-mediated recycling of IgG and albumin in human: pathophysiology and therapeutic implications using a simplified mechanism-based model. Clin Immunol 2007;122:146-55. [Crossref] [PubMed]
- Simister NE, Rees AR. Isolation and characterization of an Fc receptor from neonatal rat small intestine. Eur J Immunol 1985;15:733-8. [Crossref] [PubMed]
- Ghetie V, Ward ES. FcRn: the MHC class I-related receptor that is more than an IgG transporter. Immunol Today 1997;18:592-8. [Crossref] [PubMed]
- Ghetie V, Hubbard JG, Kim JK, et al. Abnormally short serum half‐lives of IgG in β2‐microglobulin‐deficient mice. Eur J Immunol 1996;26:690-6. [Crossref] [PubMed]
- Rodewald R, Kraehenbuhl JP. Receptor-mediated transport of IgG. J Cell Biol 1984;99:159s. [Crossref] [PubMed]
- Leach JL, Sedmak DD, Osborne JM, et al. Isolation from human placenta of the IgG transporter, FcRn, and localization to the syncytiotrophoblast: implications for maternal-fetal antibody transport. J Immunol 1996;157:3317-22. [PubMed]
- Latvala S, Jacobsen B, Otteneder MB, et al. Distribution of FcRn across species and tissues. J Histochem Cytochem 2017;65:321-33. [Crossref] [PubMed]
- Akilesh S, Christianson GJ, Roopenian DC, et al. Neonatal FcR expression in bone marrow-derived cells functions to protect serum IgG from catabolism. J Immunol 2007;179:4580-8. [Crossref] [PubMed]
- Wang Y, Tian Z, Thirumalai D, et al. Neonatal Fc receptor (FcRn): a novel target for therapeutic antibodies and antibody engineering. J Drug Target 2014;22:269-78. [Crossref] [PubMed]
- Idusogie EE, Presta LG, Gazzano-Santoro H, et al. Mapping of the C1q binding site on rituxan, a chimeric antibody with a human IgG1 Fc. J Immunol 2000;164:4178-84. [Crossref] [PubMed]
- Martin WL, West AP Jr, Gan L, et al. Crystal structure at 2.8 Å of an FcRn/heterodimeric Fc complex: mechanism of pH-dependent binding. Mol Cell 2001;7:867-77. [Crossref] [PubMed]
- Kim JK, Tsen MF, Ghetie V, et al. Localization of the site of the murine IgG1 molecule that is involved in binding to the murine intestinal Fc receptor. Eur J Immunol 1994;24:2429-34. [Crossref] [PubMed]
- Kim JK, Firan M, Radu CG, et al. Mapping the site on human IgG for binding of the MHC class I‐related receptor, FcRn. Eur J Immunol 1999;29:2819-25. [Crossref] [PubMed]
- Kabat EA, Te Wu T, Perry HM, et al. Sequences of proteins of immunological interest. DIANE publishing; 1992.
- Vaughn DE, Milburn CM, Penny DM, et al. Identification of critical IgG binding epitopes on the neonatal Fc receptor. J Mol Biol 1997;274:597-607. [Crossref] [PubMed]
- Raghavan M, Bonagura VR, Morrison SL, et al. Analysis of the pH dependence of the neonatal Fc receptor/immunoglobulin G interaction using antibody and receptor variants. Biochemistry 1995;34:14649-57. [Crossref] [PubMed]
- Zhou J, Johnson JE, Ghetie V, et al. Generation of mutated variants of the human form of the MHC class I-related receptor, FcRn, with increased affinity for mouse immunoglobulin G. J Mol Biol 2003;332:901-13. [Crossref] [PubMed]
- Ober RJ, Martinez C, Lai X, et al. Exocytosis of IgG as mediated by the receptor, FcRn: an analysis at the single-molecule level. Proc Natl Acad Sci U S A 2004;101:11076-81. [Crossref] [PubMed]
- Ober RJ, Martinez C, Vaccaro C, et al. Visualizing the site and dynamics of IgG salvage by the MHC class I-related receptor, FcRn. J Immunol 2004;172:2021-9. [Crossref] [PubMed]
- Prabhat P, Gan Z, Chao J, et al. Elucidation of intracellular recycling pathways leading to exocytosis of the Fc receptor, FcRn, by using multifocal plane microscopy. Proc Natl Acad Sci U S A 2007;104:5889-94. [Crossref] [PubMed]
- Ward ES, Velmurugan R, Ober RJ. Targeting FcRn for therapy: from live cell imaging to in vivo studies in mice. Immunol Lett 2014;160:158-62. [Crossref] [PubMed]
- Ward ES, Devanaboyina SC, Ober RJ. Targeting FcRn for the modulation of antibody dynamics. Mol Immunol 2015;67:131-41. [Crossref] [PubMed]
- Junghans RP, Anderson C. The protection receptor for IgG catabolism is the beta2-microglobulin-containing neonatal intestinal transport receptor. Proc Natl Acad Sci U S A 1996;93:5512-6. [Crossref] [PubMed]
- Ward ES, Zhou J, Ghetie V, et al. Evidence to support the cellular mechanism involved in serum IgG homeostasis in humans. Int Immunol 2003;15:187-95. [Crossref] [PubMed]
- Waldmann TA, Strober W, BLAESE RM. Metabolism of immunoglobulins. Progress in Immunology. Elsevier; 1971. p. 891-903.
- Andersen JT, Dalhus B, Cameron J, et al. Structure-based mutagenesis reveals the albumin-binding site of the neonatal Fc receptor. Nat Commun 2012;3:610. [Crossref] [PubMed]
- Chaudhury C, Mehnaz S, Robinson JM, et al. The major histocompatibility complex–related Fc receptor for IgG (FcRn) binds albumin and prolongs its lifespan. J Exp Med 2003;197:315-22. [Crossref] [PubMed]
- Theofilopoulos AN, Dixon FJ. Murine models of systemic lupus erythematosus. Advances in immunology. Elsevier; 1985. p. 269-390.
- Feldmann M, Brennan FM, Maini RN. Role of cytokines in rheumatoid arthritis. Annu Rev Immunol 1996;14:397-440. [Crossref] [PubMed]
- Vyse TJ, Kotzin BL. Genetic basis of systemic lupus erythematosus. Curr Opin Immunol 1996;8:843-51. [Crossref] [PubMed]
- Mathey EK, Park SB, Hughes RA, et al. Chronic inflammatory demyelinating polyradiculoneuropathy: from pathology to phenotype. J Neurol Neurosurg Psychiatry 2015;86:973-85. [Crossref] [PubMed]
- Aoyama Y, Owada MK, Kitajima Y. A pathogenic autoantibody, pemphigus vulgaris‐IgG, induces phosphorylation of desmoglein 3, and its dissociation from plakoglobin in cultured keratinocytes. Eur J Immunol 1999;29:2233-40. [Crossref] [PubMed]
- Kashiwagi H, Tomiyama Y. Pathophysiology and management of primary immune thrombocytopenia. Int J Hematol 2013;98:24-33. [Crossref] [PubMed]
- Salama A. Emerging drugs for immune thrombocytopenia (ITP). Expert Opin Emerg Drugs 2017;22:27-38. [Crossref] [PubMed]
- Chan H, Moore JC, Finch CN, et al. The IgG subclasses of platelet‐associated autoantibodies directed against platelet glycoproteins IIb/IIIa in patients with idiopathic thrombocytopenic purpura. Br J Haematol 2003;122:818-24. [Crossref] [PubMed]
- Hymes K, Schur P, Karpatkin S. Heavy-chain subclass of round antiplatelet IgG in autoimmune thrombocytopenic purpura. Blood 1980;56:84-7. [Crossref] [PubMed]
- McMillan R. Antiplatelet antibodies in chronic adult immune thrombocytopenic purpura: assays and epitopes. J Pediatr Hematol Oncol 2003;25 Suppl 1:S57-61. [Crossref] [PubMed]
- McMillan R. Antiplatelet antibodies in chronic immune thrombocytopenia and their role in platelet destruction and defective platelet production. Hematol Oncol Clin North Am 2009;23:1163-75. [Crossref] [PubMed]
- Cuker A, Neunert CE. How I treat refractory immune thrombocytopenia. Blood 2016;128:1547-54. [Crossref] [PubMed]
- Buskard N, Rock G, Nair R, et al. The Canadian experience using plasma exchange for immune thrombocytopenic purpura. Transfusion science 1998;19:295-300. [Crossref] [PubMed]
- Marder VJ, Nusbacher J, Anderson F. One‐year follow‐up of plasma exchange therapy in 14 patients with idiopathic thrombocytopenic purpura. Transfusion 1981;21:291-8. [Crossref] [PubMed]
- Kazatchkine MD, Kaveri SV. Immunomodulation of autoimmune and inflammatory diseases with intravenous immune globulin. N Engl J Med 2001;345:747-55. [Crossref] [PubMed]
- Imbach P, d'Apuzzo V, Hirt A, et al. High-dose intravenous gammaglobulin for idiopathic thrombocytopenic purpura in childhood. lancet 1981;317:1228-31.
- Newland AC, Treleaven J, Minchinton R, et al. High-dose intravenous IgG in adults with autoimmune thrombocytopenia. Lancet 1983;1:84-7. [Crossref] [PubMed]
- Witkowski M, Witkowska M, Robak T. Autoimmune thrombocytopenia: current treatment options in adults with a focus on novel drugs. Eur J Haematol 2019;103:531-41. [Crossref] [PubMed]
- Godeau B, Caulier MT, Decuypere L, et al. Intravenous immunoglobulin for adults with autoimmune thrombocytopenic purpura: results of a randomized trial comparing 0.5 and 1 g/kg bw. Br J Haematol 1999;107:716-9. [Crossref] [PubMed]
- Salama A, Mueller-Eckhardt C, Kiefel V. Effect of intravenous immunoglobulin in immune thrombocytopenia: Competitive inhibition of reticuloendothelial system function by sequestration of autologous red blood cells? Lancet 1983;2:193-5. [Crossref] [PubMed]
- Jin F, Balthasar JP. Mechanisms of intravenous immunoglobulin action in immune thrombocytopenic purpura. Hum Immunol 2005;66:403-10. [Crossref] [PubMed]
- Andersen JT, Justesen S, Berntzen G, et al. A strategy for bacterial production of a soluble functional human neonatal Fc receptor. J Immunol Methods 2008;331:39-49. [Crossref] [PubMed]
- Sesarman A, Vidarsson G, Sitaru C. The neonatal Fc receptor as therapeutic target in IgG-mediated autoimmune diseases. Cell Mol Life Sci 2010;67:2533-50. [Crossref] [PubMed]
- Vaccaro C, Zhou J, Ober RJ, et al. Engineering the Fc region of immunoglobulin G to modulate in vivo antibody levels. Nat Biotechnol 2005;23:1283-8. [Crossref] [PubMed]
- Kenniston JA, Taylor BM, Conley GP, et al. Structural basis for pH-insensitive inhibition of immunoglobulin G recycling by an anti-neonatal Fc receptor antibody. J Biol Chem 2017;292:17449-60. [Crossref] [PubMed]
- Challa DK, Bussmeyer U, Khan T, et al., editors. Autoantibody depletion ameliorates disease in murine experimental autoimmune encephalomyelitis. MAbs; 2013: Taylor & Francis.
- Newland AC, Sánchez‐González B, Rejtő L, et al. Phase 2 study of efgartigimod, a novel FcRn antagonist, in adult patients with primary immune thrombocytopenia. Am J Hematol 2020;95:178-87. [Crossref] [PubMed]
- Howard JF, Bril V, Burns TM, et al. Randomized phase 2 study of FcRn antagonist efgartigimod in generalized myasthenia gravis. Neurology 2019;92:e2661-73. [Crossref] [PubMed]
- Kaplan AA. Therapeutic plasma exchange: a technical and operational review. J Clin Apher 2013;28:3-10. [Crossref] [PubMed]
- Ohkubo A, Okado T, Kurashima N, et al. Removal characteristics of immunoglobulin G subclasses by conventional plasma exchange and selective plasma exchange. Ther Apher Dial 2015;19:361-6. [Crossref] [PubMed]
- Robak T, Kaźmierczak M, Jarque I, et al. Phase 2 multiple-dose study of an FcRn inhibitor, rozanolixizumab, in Patients with Primary Immune Thrombocytopenia. Blood Adv 2020;4:4136-46. [Crossref] [PubMed]
- Huda R. New Approaches to Targeting B Cells for Myasthenia Gravis Therapy. Frontiers in Immunology 2020;11:240. [Crossref] [PubMed]
- Filomena CA, Filomena AP, Hudock J, et al. Evaluation of serum immunoglobulins by protein electrophoresis and rate nephelometry before and after therapeutic plasma exchange. American journal of clinical pathology 1992;98:243-8. [Crossref] [PubMed]
- Ling LE, Hillson JL, Tiessen RG, et al. M281, an Anti-FcRn Antibody: Pharmacodynamics, Pharmacokinetics, and Safety Across the Full Range of IgG Reduction in a First-in-Human Study. Clin Pharmacol Ther 2019;105:1031-9. [Crossref] [PubMed]
- Collins J, Jones L, Snyder M, et al. RVT-1401, A Novel Anti-FcRn Monoclonal Antibody, Is Well Tolerated in Healthy Subjects and Reduces Plasma IgG Following Subcutaneous or Intravenous Administration (P5. 2-079). AAN Enterprises; 2019.
- Oganesyan V, Damschroder MM, Cook KE, et al. Structural insights into neonatal Fc receptor-based recycling mechanisms. J Biol Chem 2014;289:7812-24. [Crossref] [PubMed]
- Weflen AW, Baier N, Tang QJ, et al. Multivalent immune complexes divert FcRn to lysosomes by exclusion from recycling sorting tubules. Mol Biol Cell 2013;24:2398-405. [Crossref] [PubMed]
- Baker K, Rath T, Flak MB, et al. Neonatal Fc receptor expression in dendritic cells mediates protective immunity against colorectal cancer. Immunity 2013;39:1095-107. [Crossref] [PubMed]
- Blumberg LJ, Humphries JE, Jones SD, et al. Blocking FcRn in humans reduces circulating IgG levels and inhibits IgG immune complex-mediated immune responses. Sci Adv 2019;5:eaax9586. [Crossref] [PubMed]
- Gable KL, Guptill JT. Antagonism of the Neonatal Fc Receptor as an Emerging Treatment for Myasthenia Gravis. Front Immunol 2020;10:3052. [Crossref] [PubMed]
- Suntharalingam G, Perry MR, Ward S, et al. Cytokine storm in a phase 1 trial of the anti-CD28 monoclonal antibody TGN1412. N Engl J Med 2006;355:1018-28. [Crossref] [PubMed]
- Dall'Acqua WF, Woods RM, Ward ES, et al. Increasing the affinity of a human IgG1 for the neonatal Fc receptor: biological consequences. J Immunol 2002;169:5171-80. [Crossref] [PubMed]
Cite this article as: Newland AC, McDonald V. FcRn antagonists in ITP. Ann Blood 2021;6:6.