Red blood cell alloimmunization and sickle cell disease: a narrative review on antibody induction
Introduction
People living with sickle cell disease (SCD) have the highest prevalence of red blood cell (RBC) alloimmunization of any studied population (1). Up to 40–50% of transfused people with SCD form RBC alloantibodies over their lifetimes (2-4), compared to fewer than 5% of the “general” transfused population (2,5). Potential reasons for this prevalence rate in people with SCD include a relatively high lifetime RBC transfusion burden, RH genetic diversity, and immune system considerations, among others. However, this prevalence is higher than that observed in other frequently transfused patient populations, including those with thalassemia major (6) or myelodysplasia (7).
RBC alloantibodies may be clinically significant in transfusion and pregnancy situations, potentially leading to hemolytic reactions or hemolytic disease of the fetus and newborn. Aside from hemolytic risks, these antibodies may make locating compatible RBC units for future transfusion difficult and at times impossible. Notably, RBC alloantibodies in people with SCD have been shown to be associated with decreased overall survival (8); related morbidity/mortality is likely under-reported (9). Besides forming RBC alloantibodies, people with SCD also readily form RBC autoantibodies as well as HLA alloantibodies (10). RBC alloantibody evanescence, or the disappearance of antibodies below a threshold detectable by the transfusion service, makes future RBC transfusion particularly dangerous as a seemingly compatible RBC unit may result in a delayed hemolytic reaction (11). Emerging literature suggests such evanescence may be more common in people with SCD than in others (12). A complication of delayed hemolytic transfusion reactions that occurs at particularly high frequency in people with SCD is bystander hemolysis, or the destruction of self-RBCs; this complication is reviewed separately.
The goal of this manuscript is to review existing data on factors influencing RBC alloantibody induction in people with SCD with a focus on inflammation and other immune system considerations, from the bench to the bedside. This article is presented in accordance with the narrative review checklist (available at http://dx.doi.org/10.21037/aob-2020-scd-01).
General immune system considerations in SCD: a broad overview
SCD impacts many aspects of the immune system, from innate to adaptive immunity. Outside of transfusion medicine, the most recognized consequence of immune dysregulation in SCD is an increased risk of infection; infection has historically been a leading cause of morbidity and mortality world-wide in people with SCD (13). Along these same lines, responses to vaccines appear to be less sustained in people with SCD (14-17). Although hyposplenism presumably impacts the risk of infection as well as vaccine responses, it is also likely that alterations in white blood cell (WBC) subsets (18), platelets, RBCs, and complement are involved to some extent. Elevated WBC counts were described decades ago in people living with SCD (19). Activated neutrophils (20) from people with SCD have been described to be associated with disease complications such as pain crises (21) and they also contribute to neutrophil extracellular traps (NETs) (22). Thrombocytosis in people with SCD contributes to the inflammatory milieu (23), with people with hemoglobin (Hgb) SS disease having higher platelet counts than those with sickle cell trait or those with Hgb AA (24-26). Further, people with SCD also have high levels of soluble CD40L (27) and baseline platelet activation (28-30). The generation of free heme from ongoing RBC breakdown activates the alternative pathway of complement (31,32) and increased levels of complement C3-C5 have been noted in people with SCD compared to controls (33).
Inflammation and RBC alloantibody induction in SCD: a broad overview
In addition to antigenic differences between donor RBCs and recipient RBCs, the inflammatory status of the recipient at the time of RBC exposure likely impacts to some extent whether a recipient may become alloimmunized or not. People transfused in their baseline states of health, for example, are thought to be less likely to become alloimmunized than people transfused in a state of inflammation (34). Having acute chest syndrome or a pain crisis at the time of a RBC transfusion is a risk factor for becoming alloimmunized (35), as is having a viral illness (36) or other inflammatory disorder (37,38). Whereas transfusion can be avoided in some inflammatory situations, in others (including acute chest syndrome) it is considered a first-line therapy (39). Given the short half-life of RBCs in people with SCD, for example, free heme is continuously being generated and thus transfusing around “chronic inflammation” associated with free heme is not possible. The impact of free heme on immune dysregulation has been reviewed by Yazdanbakhsh et al. (40), with potential effects on the antigen presenting cell/CD4+ T-cell axis.
Inflammation and RBC alloantibody induction in murine models
Murine models have allowed inflammation-related variables to be dissected in ways simply not feasible in humans. For example, murine models can be designed such that the only non-self antigen in a transfused RBC unit is the KEL glycoprotein, the human glycophorin A antigen, or the triple fusion protein HOD (hen egg lysozyme, ovalbumin, human Duffyb). The KEL and HOD mice were generated using a B-globin promotor (41,42), whereas the hGPA mice were generated using a BAC construct (43). The toll-like receptor 3 agonist [poly (I:C)], a double stranded RNA that mimics viral-like inflammation, is the form of inflammation that most consistently increases RBC alloimmunization in murine models (44-48). In some models, recipient treatment with poly (I:C) takes animals that would otherwise not form RBC alloantibodies and turns them into alloimmunized responders; in other models, poly (I:C) increases the magnitude of the alloantibody responses. For example, C57BL/6 wild type recipients of murine RBCs expressing the KEL glycoprotein transfused in the presence of an adjuvant like poly (I:C) develop anti-KEL glycoprotein alloantibodies within days, with IgM forming first and then IgG developing days later (44). These anti-KEL antibodies are clinically significant and result in hemolysis of incompatible RBCs (49) as well as hemolytic disease of the fetus and newborn (50). Over the past few years multiple different KEL donor models have been studied, with transfusion of RBCs from one strain requiring an adjuvant to lead to an alloantibody response (51) and with transfusion of RBCs from a different strain being able to generate a moderate antibody responses without an adjuvant but having a higher magnitude of a response with an adjuvant (41). Likewise, recipients of murine hGPA RBCs develop anti-hGPA alloantibodies if transfused in the presence of poly (I:C) (45) or CpG; these antibodies are also clinically significant and lead to hemolysis of incompatible RBCs (52). Finally, recipients of murine HOD RBCs develop anti-HOD alloantibodies, with recipients pretreated with poly (I:C) generating a higher magnitude of anti-HOD antibodies (53). Besides poly (I:C), other forms of inflammation have been shown to impact RBC alloimmunization in murine models. For example, the toll-like receptor 4 (TLR4) agonist and bacterial endotoxin-like stimulus lipopolysaccharide increases alloimmunization rates in one model (47). Additionally, the toll-like receptor 9 (TLR9) agonist CpG, when mixed in with RBCs to be transfused, has been described by Yu et al. to increase RBC alloimmunization to murine RBCs expressing the hGPA antigen (54,55).
Outside of transfusion medicine, type 1 interferon (e.g., IFNα/β) is an important regulator of antiviral immunity and autoimmune pathology, with signal transduction through the interferon receptor (IFNAR) resulting in the expression of numerous interferon-stimulated genes (ISGs) that inhibit viral replication and dissemination (56). IFNα/β has been implicated in the pathogenesis of multiple autoimmune diseases, including rheumatoid arthritis, dermatomyositis, scleroderma, system lupus erythematosus, and Sjögren’s syndrome (57-61). Further, multiple studies have shown that IFNα/β promotes adjuvant-induced antibody responses to soluble antigens by increasing activation of dendritic cells and lymphocytes (62-64). As autoimmune diseases are also associated with high prevalence rates of RBC alloimmunization (2,38,65), Gibb et al. hypothesized that IFNα/β may play a role in RBC alloimmunization. In fact, treatment with exogenous type 1 IFN has a similar adjuvant effect as treatment with poly (I:C) (51), and animals lacking functional type 1 interferon receptors (IFNAR knock out animals) do not form RBC alloantibodies. Further, animals that cannot readily generate IFNα/β (e.g. animals lacking interferon regulatory factor (IRF) 3/7 as well as those lacking the upstream poly (I:C) receptor mitochondrial antiviral signaling pathway) also do not become alloimmunized following poly (I:C) treatment. Thus, IFNα/β plays a critical role in the way that poly (I:C) enhances alloimmunization in the murine models studied to date. Recently, recipient influenza infection has also been shown to exert a similar effect as poly (I:C) on RBC alloantibody induction in a murine model (66) and recipient treatment with exogenous type 1 IFN (IFNα) has been shown to have a similar adjuvant effect as poly (I:C) treatment (51).
To date, only a single study has been published investigating RBC alloimmunization induction in murine models of SCD. RBCs expressing the HOD antigen, transfused into animals with SCD (Hgb SS), did not result in an increase in responsiveness or in the magnitude of responsiveness compared with those transfused into animals with Hgb AS or Hgb AA (67). Murine models of SCD, summarized by Lizarralde-Iragorri and Shet (68), are just that; as such, it is not entirely clear how to interpret these data in the context of human SCD.
Characteristic of cell subsets as related to alloimmunization status in SCD
T-cells
With the assumption that most RBC alloantibody responses are CD4+ T-cell dependent in humans, descriptive studies have evaluated overall T-cell subsets in people with SCD with or without RBC alloantibodies. In general, people with SCD have been reported to have a lower percentage of CD4+ T-cells of all WBCs compared to race-matched controls, given the relatively higher percentage of monocytes and neutrophils. However, absolute numbers of CD4+ T-cells tend to be high in people with SCD given baseline leukocytosis (18,69,70). RBC alloimmunized children with SCD have been reported to have a higher percentage of circulating memory CD4+ T-cells relative to naïve CD4+ T-cells compared to children without antibodies (4); these findings have been replicated in adults with SCD (71). Neither of these studies evaluated antigen specific T-cells, however. Another study reported a relatively larger percentage of central memory CD4+ T-cells in an RBC alloimmunized population with SCD compared to healthy controls but not compared to a non-alloimmunized population with SCD (72).
Circulating T-follicular helper (Tfh) or Tfh-like cells (73) presumably play a role in alloantibody induction, at least for T-cell dependent antigens. Studies in autoimmune disease have reported a larger percentage of “highly functional” Tfh-like cells, whereas other studies have focused on the imbalance between Tfh and regulatory T-cells (Tfr) (74). Somewhat surprisingly, one SCD study reported a higher percentage of Tfh cells expressing CXCR5 and PD1 in non-alloimmunized subjects with SCD compared to alloimmunized subjects (72) and another study found no difference between those circulating populations (71). On the other hand, a study reported the presence of TIGIT (the T-cell immunoreceptor with IgG and immunoreceptor tyrosine-based inhibitory domains) positive Tfh cells expressing higher levels of CD40L in alloimmunized subjects with SCD (75), with such cells known to provide potent B-cell help.
B-cells
Extensive studies of B-cells in people with SCD are lacking, though modest increases in B-cell percentages of WBCs in general have been reported compared to controls (70). One study reported that regulatory B-cells from alloimmunized people with SCD have an impaired ability to inhibit monocyte proinflammatory cytokine production (76). Loss of IgM-secreting CD27+IgMhighIgDlow memory B cells has also been described in people with SCD (18), leading one to ask whether this plays any role in the relatively rapid evanescence of RBC alloantibodies (12). Although the relevance to RBC alloimmunization is not clear, a study in mice with SCD has described defective B-1b and B-2 populations, suggesting that these findings might play a role in impaired immunity to the pneumococcal vaccine (77).
Monocytes and antigen presenting cells
Multiple studies have investigated the role that monocytes may play in RBC alloimmunization (as well as other complications of SCD). Work completed by Zhong et al. has found that monocytes from people with alloantibodies express lower levels of heme oxygenase 1 (HO-1) compared with those from people without alloantibodies (78). Further, dendritic cells from alloimmunized people with SCD have been reported to be relatively refractory to the effect of free heme to down-regulate the CD83 marker; such down-regulation decreases proinflammatory effector T-cells (79). This resistance to free heme has been reported to be dependent on TLR4, with DCs from alloimmunized people with SCD having an impaired ability to inhibit NF-κB.
Given the role that monocytes play in immune responses, a recent study hypothesized that FcγR1 (CD64) expression on monocytes may be associated with RBC alloimmunization status in people with SCD. Contrary to the hypothesis, lower CD64 expression was observed on classical (CD64+CD14highCD16low) and intermediate circulating monocytes (CD64+CD14highCD16high) in alloimmunized people with SCD compared to non-alloimmunized people (80).
In murine models, dendritic cells have been shown to play a key role in alloimmune responses to transfused RBCs expressing model blood group antigens, with transient depletion of conventional dendritic cells abrogating T and B-cell responses (81). Whereas multiple types of splenic dendritic cells have been shown to phagocytose transfused RBCs, only bridging channel (33D1+) dendritic cells were shown to be required for RBC alloimmunization (81).
Neutrophils
Though not conventionally considered antigen presenting cells, neutrophils have recently been shown to phagocytose RBCs and then to acquire an antigen presenting cell phenotype (82,83). Neutrophils have also been studied in other autoimmune disease such as systemic lupus erythematosus (SLE) in relation to their contribution to disease in general. While dendritic cells are more traditionally known for type 1 IFN production, bone marrow neutrophils have been shown to be drivers of type 1 IFN production and activation in SLE (84) (a disease also associated with a high prevalence rate of RBC alloimmunization). In a recent publication, Hermand et al. reported that neutrophils from a small cohort of people with SCD had high expression of proteins belonging to the type 1 IFN response pathway (85). Serum levels of IFNα were found to be 10–1,000 fold higher in about half of all people with SCD compared to controls, with increases in interferon signaling proteins (ISG15, IFIT1, MX1, STAT1, and STAT2) reported even in people with a normal serum level of IFNα (85). The relationship of these findings to RBC alloimmunization status remains to be evaluated.
Platelets
Platelets, best known for their role in hemostasis and thrombosis, are increasingly being appreciated to participate in innate and adaptive immunity (23,86-91). To date, however, the role of platelets in RBC alloantibody formation in the general population has not been considered beyond one case series investigating febrile reactions to transfused platelets and the role of that fever on subsequent RBC alloimmunization (92). Platelets in people with SCD were first described to be activated more than a quarter of a decade ago (30), and drugs that target P-selectin have shown recent success in mitigating painful crises (93). Although studies have shown higher levels of CD40L and CD62p on platelets from people with SCD than from controls (28,29), only one small study to date has considered the role that endogenous platelets in people with SCD may play in the high prevalence rate of RBC alloimmunization (94). One observation worthy of brief mention is that people on chronic RBC erythrocytapheresis have been described to form fewer new RBC alloantibodies than those on simple transfusion therapy (95,96), despite exposure to significantly more RBC donors. The erythrocytapheresis procedure itself transiently removes about 50% of circulating platelets, making one wonder if endogenous platelets do in fact play a role in alloimmunization in humans. Future studies in this area are awaited.
Microparticles
Extracellular vesicles, including microparticles, are a topic of interest in SCD. However, the potential role of microparticles in RBC alloantibody development is unclear to date. Nader et al. (97) and others (98,99) have reviewed the existing literature on microparticles and SCD. Generated primarily from RBCs and platelets, the number of microparticles is significantly higher in people with SCD compared with healthy controls, and these numbers increase further with increasing degrees of hemolysis and with vaso-occlusion. Microparticles can promote endothelial cell activation and can increase the adhesion of neutrophils to endothelial cells (99,100). Of note, hydroxyurea use has recently been shown to decrease phosphatidylserine expression and the pro-inflammatory properties of microparticles (99). Microparticles also activate complement (101). Future studies of microparticles as “biomarkers” or “bioeffectors” in SCD (99) are anxiously awaited.
Cytokines
Polymorphisms of tumor necrosis factor-α and interleukin-1β have been reported as being over-represented in alloimmunized compared to non-alloimmunized people with SCD living in Brazil (102). In addition, interleukin-6 receptor signaling has been shown to impact RBC alloimmunization in a murine model (103). Overall serum levels of multiple cytokines have not been correlated with a history of RBC alloimmunization in at least two studies of people with SCD (80,104); studies of serum cytokine profiles at the time of new antibody formation, though logistically difficult to coordinate, might be more informative.
Complement
Constitutive complement activation is present with SCD, with the alternative pathway shown to be chronically activated in SCD more than 35 years ago (105). People with SCD have increased levels of complement C3, C4, and C5 compared to healthy controls (33), as well as increased erythrocyte C3 binding (106). Sickled RBCs that are dense are thought to be particularly susceptible to C5b-9 mediated lysis (107). The triad of free heme, TLR4, and P-selectin expression on endothelial cells has recently been shown to facilitate complement deposition, and then to provide a feedback loop for additional alternative complement pathway activation (108). Hyperhemolysis-mediated activation of the alternative complement pathway was described in a child with SCD with recurrent delayed hemolytic transfusion reactions (109,110). To date, no connection between RBC alloimmunization in general and the degree of hemolysis or the severity of anemia at baseline have been described, however. The question that remains unanswered at this point is whether this constitutive alternative pathway complement activation plays a role in the induction of RBC alloantibodies in humans with SCD; studies in mice have shown that complement can regulate RBC alloantibody induction in the absence of recipient CD4+ T-cells (110,111).
Other pathways or cells of potential interest in humans with relevance to RBC alloimmunization
A customized single nucleotide polymorphism (SNP) panel was utilized to evaluate 700 sequence variants in a multi-center case-control study of people with SCD living in France and The Netherlands (112). The group did not find any large-effect SNPS associated with RBC alloimmunization, though 19 SNPs that were determined to be moderately associated with RBC alloimmunization were identified in the secondary analysis. Of these, the authors concluded that rs5743618 in TLR1 was likely the most important risk factor for RBC alloimmunization of the SNPs studied, with a four-fold increased risk of alloimmunization per A-allele. A SNP in TANK (encoding the protein TRAF family member-associated NF-kappa-B activator) was also identified as being of interest. SNPs found to be associated with alloimmunization prevention included those in STAM (encoding a protein involved in downstream signaling of cytokine receptors including IL-2 and GM-CSF), STAT4, and IFNAR (112). A different group reported a regulatory locus on Chromosome 5 to be associated with RBC alloantibody formation (113), with the same group reporting no large-effect SNPs in a smaller study of alloimmunized and non-alloimmunized people with SCD (114).
Low affinity Fcγ receptors have been evaluated in the context of RBC alloimmunization, given the known role of these receptors in different autoimmune and alloimmune diseases. People with SCD living in Europe that had a particular polymorphism (the FCGR2Cnc-ORF polymorphism) were reported to have a 3-fold lower risk for RBC alloimmunization compared to people with SCD without that non-classical haplotype (115). However, this association was strongest for antigens other than Rh and K, which are among the most immunogenic. Recently, an Fcγ receptor 2B haplotype (2B.4) was shown to predict a significantly increased risk of RBC alloimmunization in people with SCD living in Brazil (116). Beyond Fcγ receptors, polymorphisms in TRIM 21 (Ro52) (117) and CD81 (118) have also been implicated in RBC alloimmunization.
HLA
For an alloantibody to develop, a person presumably must be exposed to a non-self antigen and have an HLA binding motif capable of presenting at least a portion of that non-self antigen. Variants of HLA class II, especially at the DR loci, have been described to be associated with RBC alloimmunization. Described in more detail elsewhere, multiple different HLA types have been shown to be capable of presenting portions of studied RBC antigens (119-126). Some HLA-DRB1 types have also been found to be associated with the development of multiple RBC and HLA alloantibodies (126).
Evanescence
RBC alloimmunization prevalence is presumably significantly higher than what has been reported, due in part of the phenomenon of evanescence, or antibodies falling below the level of detection by traditional blood bank methodologies. As described earlier, at least one study has reported higher degrees of RBC alloantibody evanescence in people with SCD compared with those in the general population (12). Fragmented medical care at different hospitals in the United States without shared medical records further increases the potential dangers of antibody evanescence (127). Without a shared RBC alloantibody registry or transfusion medicine database (128), this will continue to be a risk to people in the United States.
In addition to increasing the risk of delayed hemolytic transfusion reactions, evanescent antibodies may also increase the risk of bystander hemolysis (or destruction of self RBCs). Reviewed elsewhere, bystander hemolysis is more likely to occur in people with SCD than in other patient populations and may be deadly (11). Case reports of the efficacy of eculizumab support a role of complement activation in bystander hemolysis (110,129).
Blood donor or donor unit considerations
This review has focused on transfusion recipient variables. It is possible, however, that blood donor or blood unit variables (beyond antigen expression alone) may play a role in alloimmunization. Blood donor variables to consider include any that could lead to RBC damage or premature RBC clearance, potentially including donor G6PD deficiency or sickle cell trait (130-132); standard of care for transfusion of patients with SCD includes selecting donor units negative for sickle cell trait. In addition to free heme, unit factors that could impact the recipient’s immune system include residual WBCs, platelets, microparticles, cytokines, or other damage-associated molecular patterns (133-135). The storage duration of a RBC unit may also impact the recipient’s immune response, through free heme exposure or through other pathways (136). Whether storage duration definitively impacts alloimmunization in people in general or with SCD remains to be determined (137-140).
How to prevent RBC alloimmunization?
This manuscript has described cells and pathways that may contribute to RBC alloimmunization (Figure 1), but what can be done to prevent alloimmunization? The short response is that this manuscript has raised more questions than it will be able to provide answers to. Judicious transfusion remains the leading strategy to prevent RBC alloimmunization, though many times transfusion cannot be avoided. Matching for blood group antigens such as C/c, E/e, and K is recommended for people with SCD (39), and phenotype as well as genotype matching is discussed in more detail elsewhere. Suppression of antibody formation through medication use has not been studied extensively, but patients receiving steroids or chemotherapy for other indications are less likely to form new alloantibodies (141). Strategies to mitigate or even prevent delayed hemolytic transfusion reactions with bystander hemolysis are actively being studied (39,129,142).
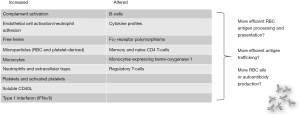
Conclusions
Although much has been learned about factors that influence the induction of RBC alloantibodies in animal models and in humans over the past few decades, much remains to be learned. It is likely that many if not most RBC alloantibodies are never identified or reported; as such, studies looking to identify risk factors for alloantibody development are hampered by the phenomenon of evanescence. Future studies incorporating basic science findings with clinical observations will help to advance our knowledge of RBC alloantibody induction; a better understanding is necessary for optimal resource utilization to aid with the development of novel strategies for antibody prevention.
Acknowledgments
Funding: This work was supported in part by HL132819 and HL132951 from the NIH to JEH.
Footnote
Provenance and Peer Review: This article was commissioned by the Guest Editor (Carla Luana Dinardo) for the series “Sickle Cell Disease” published in Annals of Blood. The article has undergone external peer review.
Reporting Checklist: The author has completed the Narrative Review reporting checklist. Available at http://dx.doi.org/10.21037/aob-2020-scd-01
Conflicts of Interest: The author has completed the ICMJE uniform disclosure form (available at http://dx.doi.org/10.21037/aob-2020-scd-01). The series “Sickle Cell Disease” was commissioned by the editorial office without any funding or sponsorship. The author has no other conflicts of interest to declare.
Ethical Statement: The author is accountable for all aspects of the manuscript and ensure that questions related to the accuracy or integrity of any part of the work are appropriately investigated and resolved.
Open Access Statement: This is an Open Access article distributed in accordance with the Creative Commons Attribution-NonCommercial-NoDerivs 4.0 International License (CC BY-NC-ND 4.0), which permits the non-commercial replication and distribution of the article with the strict proviso that no changes or edits are made and the original work is properly cited (including links to both the formal publication through the relevant DOI and the license). See: https://creativecommons.org/licenses/by-nc-nd/4.0/.
References
- Tormey CA, Hendrickson JE. Transfusion-related red blood cell alloantibodies: induction and consequences. Blood 2019;133:1821-30. [Crossref] [PubMed]
- Karafin MS, Westlake M, Hauser RG, et al. Risk factors for red blood cell alloimmunization in the Recipient Epidemiology and Donor Evaluation Study (REDS-III) database. Br J Haematol 2018;181:672-81. [Crossref] [PubMed]
- Chou ST, Jackson T, Vege S, et al. High prevalence of red blood cell alloimmunization in sickle cell disease despite transfusion from Rh-matched minority donors. Blood 2013;122:1062-71. [Crossref] [PubMed]
- Nickel RS, Horan JT, Fasano RM, et al. Immunophenotypic parameters and RBC alloimmunization in children with sickle cell disease on chronic transfusion. Am J Hematol 2015;90:1135-41. [Crossref] [PubMed]
- Redman M, Regan F, Contreras M. A prospective study of the incidence of red cell allo-immunisation following transfusion. Vox Sanguinis 1996;71:216-20. [Crossref] [PubMed]
- Vichinsky E, Neumayr L, Trimble S, et al. Transfusion complications in thalassemia patients: a report from the Centers for Disease Control and Prevention (CME). Transfusion 2014;54:972-81; quiz 1. [Crossref] [PubMed]
- Singhal D, Kutyna MM, Chhetri R, et al. Red cell alloimmunization is associated with development of autoantibodies and increased red cell transfusion requirements in myelodysplastic syndrome. Haematologica 2017;102:2021-9. [Crossref] [PubMed]
- Telen MJ, Afenyi-Annan A, Garrett ME, et al. Alloimmunization in sickle cell disease: changing antibody specificities and association with chronic pain and decreased survival. Transfusion 2015;55:1378-87. [Crossref] [PubMed]
- Nickel RS, Hendrickson JE, Fasano RM, et al. Impact of red blood cell alloimmunization on sickle cell disease mortality: a case series. Transfusion 2016;56:107-14. [Crossref] [PubMed]
- Nickel RS, Hendrickson JE, Yee MM, et al. Red blood cell transfusions are associated with HLA class I but not H-Y alloantibodies in children with sickle cell disease. Br J Haematol 2015;170:247-56. [Crossref] [PubMed]
- Siddon AJ, Kenney BC, Hendrickson JE, et al. Delayed haemolytic and serologic transfusion reactions: pathophysiology, treatment and prevention. Curr Opin Hematol 2018;25:459-67. [Crossref] [PubMed]
- Williams LA 3rd, Lorenz RG, Tahir A, et al. High Percentage of Evanescent Red Cell Antibodies in Patients with Sickle Cell Disease Highlights Need for a National Antibody Database. South Med J 2016;109:588-91. [Crossref] [PubMed]
- Booth C, Inusa B, Obaro SK. Infection in sickle cell disease: a review. Int J Infect Dis 2010;14:e2-e12. [Crossref] [PubMed]
- Hord J, Windsor B, Koehler M, et al. Diminished antibody response to hepatitis B immunization in children with sickle cell disease. J Pediatr Hematol Oncol 2002;24:548-9. [Crossref] [PubMed]
- Purohit S, Alvarez O, O'Brien R, et al. Durable immune response to inactivated H1N1 vaccine is less likely in children with sickle cell anemia receiving chronic transfusions. Pediatr Blood Cancer 2012;59:1280-3. [Crossref] [PubMed]
- Barrett DJ, Ammann AJ. Pneumococcal vaccine in sickle cell disease: IgG and IgM antibody response. Rev Infect Dis 1981;3:S179-82. [Crossref] [PubMed]
- Le Ng X, Alikhan M, Stark JM, et al. Comparison of pneumococcal vaccination response in children with sickle cell disease: HbSS and HbSC. Allergol Immunopathol (Madr) 2019;47:564-9. [Crossref] [PubMed]
- Balandya E, Reynolds T, Obaro S, et al. Alteration of lymphocyte phenotype and function in sickle cell anemia: Implications for vaccine responses. Am J Hematol 2016;91:938-46. [Crossref] [PubMed]
- West MS, Wethers D, Smith J, et al. Laboratory profile of sickle cell disease: a cross-sectional analysis. The Cooperative Study of Sickle Cell Disease. J Clin Epidemiol 1992;45:893-909. [Crossref] [PubMed]
- Lard LR, Mul FP, de Haas M, et al. Neutrophil activation in sickle cell disease. J Leukoc Biol 1999;66:411-5. [Crossref] [PubMed]
- Anyaegbu CC, Okpala IE, Akren'Ova YA, et al. Peripheral blood neutrophil count and candidacidal activity correlate with the clinical severity of sickle cell anaemia (SCA). Eur J Haematol 1998;60:267-8. [Crossref] [PubMed]
- Zhang D, Xu C, Manwani D, et al. Neutrophils, platelets, and inflammatory pathways at the nexus of sickle cell disease pathophysiology. Blood 2016;127:801-9. [Crossref] [PubMed]
- Kapur R, Semple JW. Platelets as immune-sensing cells. Blood Adv 2016;1:10-4. [Crossref] [PubMed]
- Antwi-Baffour S, Kyeremeh R, Annison L. Severity of Anaemia Has Corresponding Effects on Coagulation Parameters of Sickle Cell Disease Patients. Diseases 2019;7:59. [Crossref] [PubMed]
- Akinbami A, Dosunmu A, Adediran A, et al. Haematological values in homozygous sickle cell disease in steady state and haemoglobin phenotypes AA controls in Lagos, Nigeria. BMC Res Notes 2012;5:396. [Crossref] [PubMed]
- Chinawa JM, Emodi IJ, Ikefuna AN, et al. Coagulation profile of children with sickle cell anemia in steady state and crisis attending the university of Nigeria teaching hospital, Ituku-Ozalla, Enugu. Niger J Clin Pract 2013;16:159-63. [PubMed]
- Lee SP, Ataga KI, Orringer EP, et al. Biologically active CD40 ligand is elevated in sickle cell anemia: potential role for platelet-mediated inflammation. Arterioscler Thromb Vasc Biol 2006;26:1626-31. [Crossref] [PubMed]
- Inwald DP, Kirkham FJ, Peters MJ, et al. Platelet and leucocyte activation in childhood sickle cell disease: association with nocturnal hypoxaemia. Br J Haematol 2000;111:474-81. [Crossref] [PubMed]
- Frelinger AL 3rd, Jakubowski JA, Brooks JK, et al. Platelet activation and inhibition in sickle cell disease (pains) study. Platelets 2014;25:27-35. [Crossref] [PubMed]
- Wun T, Paglieroni T, Rangaswami A, et al. Platelet activation in patients with sickle cell disease. Br J Haematol 1998;100:741-9. [Crossref] [PubMed]
- Roumenina LT, Radanova M, Atanasov BP, et al. Heme interacts with c1q and inhibits the classical complement pathway. J Biol Chem 2011;286:16459-69. [Crossref] [PubMed]
- Frimat M, Tabarin F, Dimitrov JD, et al. Complement activation by heme as a secondary hit for atypical hemolytic uremic syndrome. Blood 2013;122:282-92. [Crossref] [PubMed]
- Okeke TI, P, Musa BO, Babadoko AA, et al. Complement Levels in Nigeria Patients with Sickle Cell Anaemia in the Asymptomatic State. Niger J Clin Pract 2018;21:1139-43. [PubMed]
- Hendrickson JE, Eisenbarth SC, Tormey CA. Red blood cell alloimmunization: new findings at the bench and new recommendations for the bedside. Curr Opin Hematol 2016;23:543-9. [Crossref] [PubMed]
- Fasasno RM, Booth GS, Miles MR, et al. RBC alloimmunization is influenced by inflammatory status at the time of transfusion in patients with sickle cell disease. Blood 2013;122:40. [Crossref]
- Evers D, van der Bom JG, Tijmensen J, et al. Red cell alloimmunisation in patients with different types of infections. Br J Haematol 2016;175:956-66. [Crossref] [PubMed]
- Celli R, Schulz W, Hendrickson JE, et al. A novel network analysis tool to identify relationships between disease states and risks for red blood cell alloimmunization. Vox Sang 2017;112:469-72. [Crossref] [PubMed]
- Ryder AB, Hendrickson JE, Tormey CA. Chronic inflammatory autoimmune disorders are a risk factor for red blood cell alloimmunization. Br J Haematol 2016;174:483-5. [Crossref] [PubMed]
- Chou ST, Alsawas M, Fasano RM, et al. American Society of Hematology 2020 guidelines for sickle cell disease: transfusion support. Blood Adv 2020;4:327-55. [Crossref] [PubMed]
- Yazdanbakhsh K, Shaz BH, Hillyer CD. Immune Regulation of sickle Cell Alloimmunization. ISBT Sci Ser 2017;12:248-53. [Crossref] [PubMed]
- Smith NH, Henry KL, Cadwell CM, et al. Generation of transgenic mice with antithetical KEL1 and KEL2 human blood group antigens on red blood cells. Transfusion 2012;52:2620-30. [Crossref] [PubMed]
- Zimring JC, Cadwell CM, Hendrickson JE, et al. Generation of a Novel Murine Model of RBC Immunology: The HEL-OVA-DUFFY Mouse. Transfusion 2008;48:186A.
- Auffray I, Marfatia S, de Jong K, et al. Glycophorin A dimerization and band 3 interaction during erythroid membrane biogenesis: in vivo studies in human glycophorin A transgenic mice. Blood 2001;97:2872-8. [Crossref] [PubMed]
- Stowell SR, Girard-Pierce KR, Smith NH, et al. Transfusion of murine red blood cells expressing the human KEL glycoprotein induces clinically significant alloantibodies. Transfusion 2014;54:179-89. [Crossref] [PubMed]
- Smith NH, Hod EA, Spitalnik SL, et al. Transfusion in the absence of inflammation induces antigen-specific tolerance to murine RBCs. Blood 2012;119:1566-9. [Crossref] [PubMed]
- Hendrickson JE, Desmarets M, Deshpande SS, et al. Recipient inflammation affects the frequency and magnitude of immunization to transfused red blood cells. Transfusion 2006;46:1526-36. [Crossref] [PubMed]
- Hendrickson JE, Roback JD, Hillyer CD, et al. Discrete toll like receptor agonists have differential effects on alloimmunization to red blood cells. Transfusion 2008;1869-77. [Crossref] [PubMed]
- Hendrickson JE, Chadwick TE, Roback JD, et al. Inflammation enhances consumption and presentation of transfused RBC antigens by dendritic cells. Blood 2007;110:2736-43. [Crossref] [PubMed]
- Girard-Pierce KR, Stowell SR, Smith NH, et al. A novel role for C3 in antibody-induced red blood cell clearance and antigen modulation. Blood 2013;122:1793-801. [Crossref] [PubMed]
- Stowell SR, Henry KL, Smith NH, et al. Alloantibodies to a paternally derived RBC KEL antigen lead to hemolytic disease of the fetus/newborn in a murine model. Blood 2013;122:1494-504. [Crossref] [PubMed]
- Gibb DR, Liu J, Natarajan P, et al. Type I IFN Is Necessary and Sufficient for Inflammation-Induced Red Blood Cell Alloimmunization in Mice. J Immunol 2017;199:1041-50. [Crossref] [PubMed]
- Liepkalns JS, Hod EA, Stowell SR, et al. Biphasic clearance of incompatible red blood cells through a novel mechanism requiring neither complement nor Fcgamma receptors in a murine model. Transfusion 2012;52:2631-45. [Crossref] [PubMed]
- Hendrickson JE, Hod EA, Spitalnik SL, et al. Storage of murine red blood cells enhances alloantibody responses to an erythroid-specific model antigen. Transfusion 2010;50:642-8. [Crossref] [PubMed]
- Yu J, Heck S, Yazdanbakhsh K. Prevention of red cell alloimmunization by CD25 regulatory T cells in mouse models. Am J Hematol 2007;82:691-6. [Crossref] [PubMed]
- Bao W, Yu J, Heck S, et al. Regulatory T-cell status in red cell alloimmunized responder and nonresponder mice. Blood 2009;113:5624-7. [Crossref] [PubMed]
- McNab F, Mayer-Barber K, Sher A, et al. Type I interferons in infectious disease. Nat Rev Immunol 2015;15:87-103. [Crossref] [PubMed]
- Olsen N, Sokka T, Seehorn CL, et al. A gene expression signature for recent onset rheumatoid arthritis in peripheral blood mononuclear cells. Ann Rheum Dis 2004;63:1387-92. [Crossref] [PubMed]
- Higgs BW, Liu Z, White B, et al. Patients with systemic lupus erythematosus, myositis, rheumatoid arthritis and scleroderma share activation of a common type I interferon pathway. Ann Rheum Dis 2011;70:2029-36. [Crossref] [PubMed]
- Båve U, Nordmark G, Lovgren T, et al. Activation of the type I interferon system in primary Sjogren's syndrome: a possible etiopathogenic mechanism. Arthritis Rheum 2005;52:1185-95. [Crossref] [PubMed]
- Baechler EC, Bauer JW, Slattery CA, et al. An interferon signature in the peripheral blood of dermatomyositis patients is associated with disease activity. Mol Med 2007;13:59-68. [Crossref] [PubMed]
- Assassi S, Mayes MD, Arnett FC, et al. Systemic sclerosis and lupus: points in an interferon-mediated continuum. Arthritis Rheum 2010;62:589-98. [Crossref] [PubMed]
- Le Bon A, Schiavoni G, D'Agostino G, et al. Type 1 interferons potently enhance humoral immunity and can promote isotype switching by stimulating dendritic cells in vivo. Immunity 2001;14:461-70. [Crossref] [PubMed]
- Proietti E, Bracci L, Puzelli S, et al. Type I IFN as a natural adjuvant for a protective immune response: lessons from the influenza vaccine model. J Immunol 2002;169:375-83. [Crossref] [PubMed]
- Le Bon A, Thompson C, Kamphuis E, et al. Cutting edge: enhancement of antibody responses through direct stimulation of B and T cells by type I IFN. J Immunol 2006;176:2074-8. [Crossref] [PubMed]
- Ramsey G, Smietana SJ. Multiple or uncommon red cell alloantibodies in women: association with autoimmune disease. Transfusion 1995;35:582-6. [Crossref] [PubMed]
- Liu D, Gibb DR, Escamilla-Rivera V, et al. Type 1 IFN signaling critically regulates influenza-induced alloimmunization to transfused KEL RBCs in a murine model. Transfusion 2019;59:3243-52. [Crossref] [PubMed]
- Hendrickson JE, Hod EA, Perry JR, et al. Alloimmunization to transfused HOD red blood cells is not increased in mice with sickle cell disease. Transfusion 2012;52:231-40. [Crossref] [PubMed]
- Lizarralde-Iragorri MA, Shet AS. Sickle Cell Disease: A Paradigm for Venous Thrombosis Pathophysiology. Int J Mol Sci 2020;21:5279. [Crossref] [PubMed]
- Musa BO, Onyemelukwe GC, Hambolu JO, et al. Pattern of serum cytokine expression and T-cell subsets in sickle cell disease patients in vaso-occlusive crisis. Clin Vaccine Immunol 2010;17:602-8. [Crossref] [PubMed]
- Nickel RS, Osunkwo I, Garrett A, et al. Immune parameter analysis of children with sickle cell disease on hydroxycarbamide or chronic transfusion therapy. Br J Haematol 2015;169:574-83. [Crossref] [PubMed]
- Balbuena-Merle R, Santhanakrishnan M, Devine L, et al. Characterization of circulating and cultured Tfh-like cells in sickle cell disease in relation to red blood cell alloimmunization status. Transfus Apher Sci 2020;59:102778 [Crossref] [PubMed]
- Vingert B, Tamagne M, Habibi A, et al. Phenotypic differences of CD4(+) T cells in response to red blood cell immunization in transfused sickle cell disease patients. Eur J Immunol 2015;45:1868-79. [Crossref] [PubMed]
- Crotty S. T Follicular Helper Cell Biology: A Decade of Discovery and Diseases. Immunity 2019;50:1132-48. [Crossref] [PubMed]
- Wen Y, Yang B, Lu J, et al. Imbalance of circulating CD4(+)CXCR5(+)FOXP3(+) Tfr-like cells and CD4(+)CXCR5(+)FOXP3(-) Tfh-like cells in myasthenia gravis. Neurosci Lett 2016;630:176-82. [Crossref] [PubMed]
- Godefroy E, Zhong H, Pham P, et al. TIGIT-positive circulating follicular helper T cells display robust B-cell help functions: potential role in sickle cell alloimmunization. Haematologica 2015;100:1415-25. [Crossref] [PubMed]
- Bao W, Zhong H, Manwani D, et al. Regulatory B-cell compartment in transfused alloimmunized and non-alloimmunized patients with sickle cell disease. Am J Hematol 2013;88:736-40. [Crossref] [PubMed]
- Cotte C, Szczepanek SM. Peritoneal B-1b and B-2 B-cells confer long-term protection from pneumococcal serotype 3 infection after vaccination with Prevnar-13 and are defective in sickle cell disease mice. Vaccine 2017;35:3520-2. [Crossref] [PubMed]
- Zhong H, Bao W, Friedman D, et al. Hemin controls T cell polarization in sickle cell alloimmunization. J Immunol 2014;193:102-10. [Crossref] [PubMed]
- Godefroy E, Liu Y, Shi P, et al. Altered heme-mediated modulation of dendritic cell function in sickle cell alloimmunization. Haematologica 2016;101:1028-38. [Crossref] [PubMed]
- Balbuena-Merle R, Curtis SA, Devine L, et al. Red blood cell alloimmunization is associated with lower expression of FcγR1 on monocyte subsets in patients with sickle cell disease. Transfusion 2019;59:3219-27. [Crossref] [PubMed]
- Calabro S, Gallman A, Gowthaman U, et al. Bridging channel dendritic cells induce immunity to transfused red blood cells. J Exp Med 2016;213:887-96. [Crossref] [PubMed]
- Meinderts SM, Baker G, van Wijk S, et al. Neutrophils acquire antigen-presenting cell features after phagocytosis of IgG-opsonized erythrocytes. Blood Adv 2019;3:1761-73. [Crossref] [PubMed]
- Meinderts SM, Oldenborg PA, Beuger BM, et al. Human and murine splenic neutrophils are potent phagocytes of IgG-opsonized red blood cells. Blood Adv 2017;1:875-86. [Crossref] [PubMed]
- Palanichamy A, Bauer JW, Yalavarthi S, et al. Neutrophil-mediated IFN activation in the bone marrow alters B cell development in human and murine systemic lupus erythematosus. J Immunol 2014;192:906-18. [Crossref] [PubMed]
- Hermand P, Azouzi S, Gautier EF, et al. The proteome of neutrophils in sickle cell disease reveals an unexpected activation of interferon alpha signaling pathway. Haematologica 2020;105:2851-4. [Crossref] [PubMed]
- Semple JW, Italiano JE Jr, Freedman J. Platelets and the immune continuum. Nat Rev Immunol 2011;11:264-74. [Crossref] [PubMed]
- Morrell CN, Sun H, Swaim AM, et al. Platelets an inflammatory force in transplantation. Am J Transplant 2007;7:2447-54. [Crossref] [PubMed]
- Verschoor A, Neuenhahn M, Navarini AA, et al. A platelet-mediated system for shuttling blood-borne bacteria to CD8alpha+ dendritic cells depends on glycoprotein GPIb and complement C3. Nat Immunol 2011;12:1194-201. [Crossref] [PubMed]
- Chapman LM, Aggrey AA, Field DJ, et al. Platelets present antigen in the context of MHC class I. J Immunol 2012;189:916-23. [Crossref] [PubMed]
- Zufferey A, Speck ER, Machlus KR, et al. Mature murine megakaryocytes present antigen-MHC class I molecules to T cells and transfer them to platelets. Blood Adv 2017;1:1773-85. [Crossref] [PubMed]
- Kapur R, Zufferey A, Boilard E, et al. Nouvelle cuisine: platelets served with inflammation. J Immunol 2015;194:5579-87. [Crossref] [PubMed]
- Yazer MH, Triulzi DJ, Shaz B, et al. Does a febrile reaction to platelets predispose recipients to red blood cell alloimmunization? Transfusion 2009;49:1070-5. [Crossref] [PubMed]
- Ataga KI, Kutlar A, Kanter J, et al. Crizanlizumab for the Prevention of Pain Crises in Sickle Cell Disease. N Engl J Med 2017;376:429-39. [Crossref] [PubMed]
- Davila J, Manwani D, Vasovic L, et al. A novel inflammatory role for platelets in sickle cell disease. Platelets 2015;26:726-9. [Crossref] [PubMed]
- Wahl SK, Garcia A, Hagar W, et al. Lower alloimmunization rates in pediatric sickle cell patients on chronic erythrocytapheresis compared to chronic simple transfusions. Transfusion 2012;52:2671-6. [Crossref] [PubMed]
- Godfrey GJ, Lockwood W, Kong M, et al. Antibody development in pediatric sickle cell patients undergoing erythrocytapheresis. Pediatr Blood Cancer 2010;55:1134-7. [Crossref] [PubMed]
- Nader E, Romana M, Connes P. The Red Blood Cell-Inflammation Vicious Circle in Sickle Cell Disease. Front Immunol 2020;11:454. [Crossref] [PubMed]
- Lapping-Carr G, Gemel J, Mao Y, et al. Circulating Extracellular Vesicles and Endothelial Damage in Sickle Cell Disease. Front Physiol 2020;11:1063. [Crossref] [PubMed]
- Garnier Y, Ferdinand S, Garnier M, et al. Plasma microparticles of sickle patients during crisis or taking hydroxyurea modify endothelium inflammatory properties. Blood 2020;136:247-56. [Crossref] [PubMed]
- Romana M, Connes P, Key NS. Microparticles in sickle cell disease. Clin Hemorheol Microcirc 2018;68:319-29. [Crossref] [PubMed]
- Merle NS, Grunenwald A, Rajaratnam H, et al. Intravascular hemolysis activates complement via cell-free heme and heme-loaded microvesicles. JCI Insight 2018;3:e96910 [Crossref] [PubMed]
- Sippert EÂ, Visentainer JE, Alves HV, et al. Red blood cell alloimmunization in patients with sickle cell disease: correlation with HLA and cytokine gene polymorphisms. Transfusion 2017;57:379-89. [Crossref] [PubMed]
- Arneja A, Salazar JE, Jiang W, et al. Interleukin-6 receptor-alpha signaling drives anti-RBC alloantibody production and T-follicular helper cell differentiation in a murine model of red blood cell alloimmunization. Haematologica 2016;101:e440-4. [Crossref] [PubMed]
- Tatari-Calderone Z, Fasano RM, Miles MR, et al. High multi-cytokine levels are not a predictive marker of alloimmunization in transfused sickle cell disease patients. Cytokine 2014;68:59-64. [Crossref] [PubMed]
- Chudwin DS, Korenblit AD, Kingzette M, et al. Increased activation of the alternative complement pathway in sickle cell disease. Clin Immunol Immunopathol 1985;37:93-7. [Crossref] [PubMed]
- Wang RH, Phillips G Jr, Medof ME, et al. Activation of the alternative complement pathway by exposure of phosphatidylethanolamine and phosphatidylserine on erythrocytes from sickle cell disease patients. J Clin Invest 1993;92:1326-35. [Crossref] [PubMed]
- Test ST, Woolworth VS. Defective regulation of complement by the sickle erythrocyte: evidence for a defect in control of membrane attack complex formation. Blood 1994;83:842-52. [Crossref] [PubMed]
- Merle NS, Paule R, Leon J, et al. P-selectin drives complement attack on endothelium during intravascular hemolysis in TLR-4/heme-dependent manner. Proc Natl Acad Sci U S A 2019;116:6280-5. [Crossref] [PubMed]
- Chonat S, Quarmyne MO, Bennett CM, et al. Contribution of alternative complement pathway to delayed hemolytic transfusion reaction in sickle cell disease. Haematologica 2018;103:e483-e485. [Crossref] [PubMed]
- Chonat S, Mener A, Verkerke H, et al. Role of complement in alloimmunization and hyperhemolysis. Curr Opin Hematol 2020;27:406-14. [Crossref] [PubMed]
- Mener A, Patel SR, Arthur CM, et al. Complement serves as a switch between CD4+ T cell-independent and -dependent RBC antibody responses. JCI Insight 2018;3:e121631 [Crossref] [PubMed]
- Meinderts SM, Gerritsma JJ, Sins JWR, et al. Identification of genetic biomarkers for alloimmunization in sickle cell disease. Br J Haematol 2019;186:887-99. [Crossref] [PubMed]
- Williams LM, Qi Z, Batai K, et al. A locus on chromosome 5 shows African ancestry-limited association with alloimmunization in sickle cell disease. Blood Adv 2018;2:3637-47. [Crossref] [PubMed]
- Hanchard NA, Moulds JM, Belmont JW, et al. A Genome-Wide Screen for Large-Effect Alloimmunization Susceptibility Loci among Red Blood Cell Transfusion Recipients with Sickle Cell Disease. Transfus Med Hemother 2014;41:453-61. [Crossref] [PubMed]
- Meinderts SM, Sins JWR, Fijnvandraat K, et al. Nonclassical FCGR2C haplotype is associated with protection from red blood cell alloimmunization in sickle cell disease. Blood 2017;130:2121-30. [Crossref] [PubMed]
- Costa Neto A, Santos F, Ribeiro I, et al. FcγR2B B2.4 haplotype predicts increased risk of red blood cell alloimmunization in sickle cell disease patients. Transfusion 2020;60:1573-8. [Crossref] [PubMed]
- Tatari-Calderone Z, Minniti CP, Kratovil T, et al. rs660 polymorphism in Ro52 (SSA1; TRIM21) is a marker for age-dependent tolerance induction and efficiency of alloimmunization in sickle cell disease. Mol Immunol 2009;47:64-70. [Crossref] [PubMed]
- Tatari-Calderone Z, Tamouza R, Le Bouder GP, et al. The association of CD81 polymorphisms with alloimmunization in sickle cell disease. Clin Dev Immunol 2013;2013:937846 [Crossref] [PubMed]
- Picard C, Frassati C, Basire A, et al. Positive association of DRB1 04 and DRB1 15 alleles with Fya immunization in a Southern European population. Transfusion 2009;49:2412-7. [Crossref] [PubMed]
- Chiaroni J, Dettori I, Ferrera V, et al. HLA-DRB1 polymorphism is associated with Kell immunisation. Br J Haematol 2006;132:374-8. [Crossref] [PubMed]
- Reviron D, Dettori I, Ferrera V, et al. HLA-DRB1 alleles and Jk(a) immunization. Transfusion 2005;45:956-9. [Crossref] [PubMed]
- Tatari-Calderone Z, Gordish-Dressman H, Fasano R, et al. Protective effect of HLA-DQB1 alleles against alloimmunization in patients with sickle cell disease. Hum Immunol 2016;77:35-40. [Crossref] [PubMed]
- Schonewille H, Doxiadis II, Levering WH, et al. HLA-DRB1 associations in individuals with single and multiple clinically relevant red blood cell antibodies. Transfusion 2014;54:1971-80. [Crossref] [PubMed]
- Baleotti W Jr, Ruiz MO, Fabron A Jr, et al. HLA-DRB1*07:01 allele is primarily associated with the Diego a alloimmunization in a Brazilian population. Transfusion 2014;54:2468-76. [Crossref] [PubMed]
- Chu CC, Ho HT, Lee HL, et al. Anti-"Mi(a)" immunization is associated with HLA-DRB1*0901. Transfusion 2009;49:472-8. [Crossref] [PubMed]
- Verduin EP, Brand A, van de Watering LM, et al. The HLA-DRB1*15 phenotype is associated with multiple red blood cell and HLA antibody responsiveness. Transfusion 2016;56:1849-56. [Crossref] [PubMed]
- Unni N, Peddinghaus M, Tormey CA, et al. Record fragmentation due to transfusion at multiple health care facilities: a risk factor for delayed hemolytic transfusion reactions. Transfusion 2014;54:98-103. [Crossref] [PubMed]
- Hauser RG, Hendrickson JE, Tormey CA. TRIX with treats: the considerable safety benefits of a transfusion medicine registry. Transfusion 2019;59:2489-92. [Crossref] [PubMed]
- Pirenne F, Bartolucci P, Habibi A. Management of delayed hemolytic transfusion reaction in sickle cell disease: Prevention, diagnosis, treatment. Transfus Clin Biol 2017;24:227-31. [Crossref] [PubMed]
- Francis RO, Jhang J, Hendrickson JE, et al. Frequency of glucose-6-phosphate dehydrogenase-deficient red blood cell units in a metropolitan transfusion service. Transfusion 2013;53:606-11. [Crossref] [PubMed]
- Osei-Hwedieh DO, Kanias T, Croix CS, et al. Sickle Cell Trait Increases Red Blood Cell Storage Hemolysis and Post-Transfusion Clearance in Mice. EBioMedicine 2016;11:239-48. [Crossref] [PubMed]
- Francis RO, D'Alessandro A, Eisenberger A, et al. Donor glucose-6-phosphate dehydrogenase deficiency decreases blood quality for transfusion. J Clin Invest 2020;130:2270-85. [Crossref] [PubMed]
- Land WG. Transfusion-Related Acute Lung Injury: The Work of DAMPs. Transfus Med Hemother 2013;40:3-13. [Crossref] [PubMed]
- Radwanski K, Garraud O, Cognasse F, et al. The effects of red blood cell preparation method on in vitro markers of red blood cell aging and inflammatory response. Transfusion 2013;53:3128-38. [Crossref] [PubMed]
- Thibault L, Beausejour A, de Grandmont MJ, et al. Characterization of blood components prepared from whole-blood donations after a 24-hour hold with the platelet-rich plasma method. Transfusion 2006;46:1292-9. [Crossref] [PubMed]
- Rapido F, Brittenham GM, Bandyopadhyay S, et al. Prolonged red cell storage before transfusion increases extravascular hemolysis. J Clin Invest 2017;127:375-82. [Crossref] [PubMed]
- Yazer MH, Triulzi DJ. Receipt of older RBCs does not predispose D-negative recipients to anti-D alloimmunization. Am J Clin Pathol 2010;134:443-7. [Crossref] [PubMed]
- Zalpuri S, Schonewille H, Middelburg R, et al. Effect of storage of red blood cells on alloimmunization. Transfusion 2013;53:2795-800. [Crossref] [PubMed]
- Dinardo CL, Fernandes FL, Sampaio LR, et al. Transfusion of older red blood cell units, cytokine burst and alloimmunization: a case-control study. Rev Bras Hematol Hemoter 2015;37:320-3. [Crossref] [PubMed]
- Desai PC, Deal AM, Pfaff ER, et al. Alloimmunization is associated with older age of transfused red blood cells in sickle cell disease. Am J Hematol 2015;90:691-5. [Crossref] [PubMed]
- Zalpuri S, Evers D, Zwaginga JJ, et al. Immunosuppressants and alloimmunization against red blood cell transfusions. Transfusion 2014;54:1981-7. [Crossref] [PubMed]
- Pirenne F, Yazdanbakhsh K. How I safely transfuse patients with sickle-cell disease and manage delayed hemolytic transfusion reactions. Blood 2018;131:2773-81. [Crossref] [PubMed]
Cite this article as: Hendrickson JE. Red blood cell alloimmunization and sickle cell disease: a narrative review on antibody induction. Ann Blood 2020;5:33.