Efficacy and mechanism of intravenous immunoglobulin treatment for immune thrombocytopenia in adults
Introduction
Immune thrombocytopenia (ITP) is a complex and rare autoimmune disease characterized by reduced platelet counts (peripheral blood platelet count <100×109/L) that can lead to an increased bleeding risk (1,2). ITP may be a primary condition in the absence of other underlying causes or disorders, or it may be secondary, associated with or caused by other diseases such as an infection, an autoimmune disease, or malignancy (2). ITP can be further classified according to The International ITP Working Group (IWG) as newly diagnosed ITP (acute; up until 3 months), persistent ITP (lasting 3–12 months, more prevalent in children), chronic ITP (lasting >12 months, more prevalent in adult patients) (2-4). This classification is important because patients with newly diagnosed and persistent ITP have significantly higher remission rates than patients with chronic ITP (2,5-8). Therefore, physicians usually recommend a more rigorous treatment plan for patients with chronic ITP, which is more predominant in adult patients (2).
Signs and symptoms, mainly hemorrhagic symptoms, are widely variable. While the predominant symptom is bleeding, and increased risk of bleeding poses the major clinical problem for ITP, bleeding symptoms may not always be present as the clinical presentation varies among ITP patients (2,5-10). Most of ITP patients are asymptomatic at presentation or show mild skin bruising and mucocutaneous bleeding (e.g., sporadic bruises or epistaxis), while only few patients present with severe, life-threatening bleeding, such as gastrointestinal bleeding and rarely, intracranial hemorrhage (4,11,12). Noteworthy, it has been shown that the risk of severe bleeding is not necessarily correlated with platelet counts unless the absolute platelet count is lower than 20×109/L, as other factors such as age, lifestyle and other clinical condition of the patient may play a role (12-17). Noteworthy, while there are similarities between children and adults with ITP, there is increased evidence that highlight several distinctive characteristic of adult ITP, such as higher rates of comorbidities and chronicity compared to children with ITP (18-20), and which can be valuable characteristics to further improve and personalize the diagnosis and treatment of this disease.
The diagnosis of ITP is one of exclusion (6). Thus, and in the absence of a “gold standard” diagnostic test for ITP, the diagnosis for this disorder remains clinical where history, physical examination, and laboratory testing are necessary to exclude other causes of thrombocytopenia (1,6,21,22). Currently, there are no clear cut-off diagnostic tools or laboratory tests to diagnose ITP, to predict the treatment response, or to identify the best treatment option for ITP patients. Therefore, the International Working Group and the American Society for Hematology published guidelines for the diagnosis and management of ITP (5,6,9,10). However, the existing guidelines are based on opinion of professionals and experts more than on the evidences of the studies (23,24).
Although treatment for patients with ITP should always be personalized to the individual patient (5,25), glucocorticoids (steroids) and intravenous immunoglobulin (IVIg), which are the most common therapeutic options, having replaced splenectomy, remain the initial ITP treatment for both newly diagnosed patients and chronic patients needing immediate rescue therapy (5,6). Glucocorticoids (GCs) are the conventional front-line therapy for ITP patients, typically either as prednisone or dexamethasone (5,6). Prednisone, the standard treatment recommended in practical guidelines for ITP, administrated at 1 mg/kg daily until the platelet level increases (≥50×109/L; can require several days to weeks) is considered the most common treatment strategy (5,26,27). GCs are an inexpensive treatment option, and it has been observed that about 70% of patients respond to the treatment within 1–2 weeks (28). Unfortunately, it has been indicated that about 70–90% of patients treated with GCs relapse when the treatment is stopped or even reduced (29). In addition, it has been revealed that GCs are associated with potentially severe side effects, and the detrimental effects of corticosteroids can create significant complications and reduce the quality of life of patients (6). Although IVIg is more expensive than GCs, it is better tolerated and is generally considered to be a safe therapy due to the minimal side effects (6,30,31). In addition, in contrast to conventional GCs treatment, it has been shown that IVIg induces recovery of platelet counts within a couple of days (32), demonstrating its therapeutic advantage when a rapid increase in the number of platelets is required, such as patients with a high risk of critical bleeding (6,23,29,33). IVIg is a blood product enriched with IgG antibodies that is obtained via collection and pooling of human plasma from several thousands of donors (34-38). IVIg is generally recommended for ITP patients under critical bleeding condition, as an emergency rescue procedure, and even for those not responding to GCs or cannot tolerate glucocorticoids (6,23,33). Several clinical trials have shown that IVIg (up to 1 g/kg) is an effective treatment in 70–80% of patient with ITP (39-42). The aim of this review is to focus on the efficacy and the general mechanisms of IVIg therapy used to treat adults with primary ITP. However, first we will provide an introduction into the pathogenesis of ITP to better understand the rationale of this important treatment.
Pathophysiology of ITP
ITP is an autoimmune hemorrhagic disease and its pathophysiology involves both excessive platelet destruction in the spleen and liver and insufficient platelet production in the bone marrow, resulting in low platelet counts (43-45).
Increased peripheral platelet destruction
Traditionally, platelet destruction in the spleen and/or liver, and less so in the periphery, is the main cause for low platelet counts in ITP. Antibody-coated platelets are destroyed by macrophages in the spleen and/or liver through interaction with Fc-gamma receptors (FcγR) (46) (Figure 1A). In the 1950s, Harrington infused blood from ITP patients to healthy volunteers, one of which was himself, and showed that most recipients demonstrated profound thrombocytopenia/low platelet counts (47). The transmissible causative factor in the blood serum was subsequently identified as an immunoglobulin, primarily immunoglobulin G (IgG), which was the first proof that a humoral factor is involved in ITP pathogenesis (48). Noteworthy, while the antiplatelet antibodies in ITP are primarily IgG, other immunoglobulin isotypes (IgA and IgM) can also be found (49). Anti-platelet antibodies are directed against platelet membrane glycoprotein (GP) or GP complexes mainly GPIIb/IIIa and GPIb/IX/V, and less against GPIa/IIa, IV or VI (49-52). As a result, anti-platelet antibodies targeting GPIIb/IIIa participate in platelet destruction when the platelets are opsonized by the attachment of autoantibodies to the GP, then bound to FcγRs expressed on macrophages, and phagocytosed (43,44). Following the phagocytosis, these macrophages present a platelet-derived antigen that stimulates CD4+ T cells, which can contribute in the activation of B cells (53). This activation leads to the differentiation of auto-reactive B cells into plasma cells to produce anti-platelet antibodies (53,54). While autoantibodies against platelet GP remains a major mechanism in the ITP pathogenesis, it is important to mention that these autoantibodies are not detected in almost half of patients with ITP, which suggest the involvement of other mechanisms (55). For instances, it has been shown that the immune platelet destruction in ITP is also associated with wide range of B-cell and T-cell involvement (46,56,57). In addition to removal of platelets by FcγR-mediated mechanisms, anti-GP1b/IX has an unusual activity where the antibody binds to platelets and removes sialic acid (58,59). These desialylated platelets are then removed through interaction with the Ashwell-Morell receptor on hepatocytes in the liver (60). This interaction results in production of the platelet growth factor, thrombopoietin (TPO), likely as a feed-back mechanism in an effort to produce more platelets. CD8+ cytotoxic T lymphocytes (CTLs) also plays a role in ITP and can directly kill platelets and megakaryocytes and/or induce desialylated platelets (61,62) (Figure 1A).
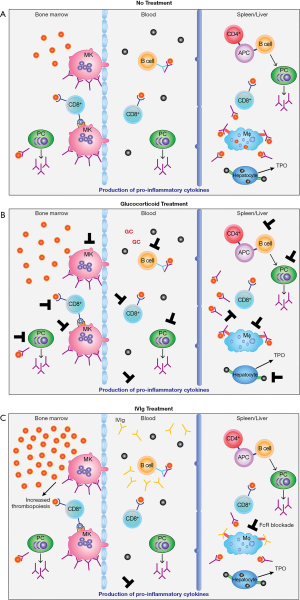
Furthermore, while genetic predispositions in ITP are uncommon, there are genetic polymorphisms in cytokines and FcγRs that may increase the risk of developing the disease in some people by participating in the initiation of the autoimmune process (63-68). Molecular mimicry may play a role in the development of cross-reactive platelet auto-antibodies as certain viral and bacterial pathogens such as human immunodeficiency virus (HIV), hepatitis C virus (HCV), varicella-zoster virus (VZV) and Helicobacter pylori (H. pylori), may express antigens/proteins that are similar to platelet GPIIIa (69-72). Interestingly, it has been shown that some peptides from these viral proteins are recognized by anti-platelet antibodies in vitro due to the similarities between the sequences of these viral proteins and platelet GPs (22). Although the absence of these bacterial and viral infections leads to remission in most patients with ITP, there is still a high variation in response rates in patients infected with H. pylori (73).
In addition, anti-platelet antibodies can mediate complement-dependent cytotoxicity, inhibit megakaryocyte function, and induce desialylation-induced platelet destruction (74-78). It has been shown that the destruction of platelets in ITP patients results in a shorter life span compared to healthy humans as confirmed by different groups using Chromium-51 labeled platelets or Indium-111 labelled platelets (79-83). This may be a result of an activated mononuclear phagocyte system in ITP patients. Noteworthy, the theory of increased platelet destruction in the spleen was also supported by the effectiveness of splenectomy in raising platelet counts in patients with ITP (81,84-86).
Decreased platelet production
Besides the platelet destruction in the circulation, mechanisms leading to inadequate platelet production in the bone marrow due to an immune response against megakaryocytes is also involved in the pathogenesis of ITP (87-90). While the immune mechanisms of insufficient platelet production in ITP remain not very well-known, there are some indications in some studies.
It has been shown that megakaryopoiesis is strongly affected in ITP as evidenced by an increase in the proportion of premature megakaryocytes and impaired development, which can be characterized by a decrease in the granularity, ploidy, cytoplasmic vacuolization and nuclear condensation, leading to significant reduction in platelet production (86,88,91). The results of radiolabeled platelet studies have revealed that the megakaryocytes in healthy individuals were able to produce 10× as many platelets as the megakaryocytes from patients with ITP during states of blood loss (45,86). A decrease in megakaryocyte maturation, abnormalities in thrombopoiesis, and inadequate platelet production rely on a specific immune response caused by autoantibodies and/or T-cell mediated megakaryocyte inhibition and destruction (46,92-94).
Additionally, it has been shown that inappropriate levels of TPO contribute to inadequate platelet production in ITP (53,95,96). TPO, the main growth factor of megakaryocytes, is predominantly and constitutively synthesized in the liver to regulate thrombopoiesis via binding and activation of its receptor, cMPL, on the megakaryocyte and platelet (97,98). Thus, the higher number of platelets released into the circulation, the lower TPO level required to stimulate the megakaryocytes to produce more platelets (99). Therefore, levels of TPO increase in the serum as an automatic compensatory response to thrombocytopenia, which has been observed by several studies (95,100-105). However, this is not the case in ITP as despite the low number of platelets in the circulation, the TPO concentration is not elevated and, instead, remains within the range of healthy individuals, which is not enough to bind to cMPL on megakaryocytes to increase platelet production in the bone marrow (53,96,104-106). Moreover, studies using electron microscopy showed that megakaryocytes from patients with ITP frequently undergo apoptosis (107,108), which may further contribute to the insufficient platelet production in these patients.
Finally, ITP has been found to present a skewed cytokine profile (109). Pro-inflammatory cytokines such as IL-2/IL-17 produced by Th1/Th17 T-cells have been documented, which can have a profound effect on T-regulatory cells and Th2 anti-inflammatory cells.
While an increase in platelet destruction and a decrease in platelet production are central aspects in the pathophysiology of ITP, patients with ITP vary with the degree of these two processes with several abnormalities and multiple components of the immune system involved (58-62,109). Therefore, this complexity and variations have led to different approaches and opened different ways for the design of specific immunotherapies to treat patients with ITP based on a case-by-case basis.
Pediatric versus adult ITP
ITP is a disorder that occurs in both adults and children with considerable differences between these two populations (110). For instance, the incidence rate of primary ITP is approximately 1.9–6.4/100,000 in children per year, and about 3.3–3.9/100,000 adults per year (2-4,111). Noteworthy, research studies and the epidemiologic data suggest that ITP that occurs in adults under the age of 65 years is more prevalent in women (ratio ~2:1) (2,4,112-117). One of the most well-documented distinctions between adult and children is that ITP in most pediatric patients tends to be acute/transient and more likely resolved eventually without any treatment. Most children undergo spontaneous remission and rarely experience active bleeding, although most of these patients still experience skin bruising and bleeding (5,6,18,19). Chronic ITP, however, is more prevalent in adult patients (~ 70–80%) and difficult to treat, as adults with ITP tend to have a higher risk of bleeding and a lower spontaneous remission rate (4,23,118). Therefore, current treatment protocols and practice guidelines for ITP are considered and developed in relationship with the clinically relevant differences between children and adult patients (5,6,23,24).
The current goals of treatment for patients with ITP are to prevent or minimize serious risk of bleeding, improve quality of life, and to achieve a safe hemostatic platelet count (generally considered to be around 20–30×109/L), which can vary between patients (119,120). Thus, considering the nature of the disease and symptoms, it has been shown that adults with ITP often undergo pharmacological therapy and splenectomy more than children (5,6,117). For newly diagnosed patients with ITP, observation and waiting without treatment is a standard approach for children whose platelet counts are above 20×109 /L with no or mild bleeding, while treatment is incorporated as standard clinical practice for adults with a platelet count below 30×109/L, which is more likely influenced by the higher risk of bleeding in adults (5,6,117,121-123). According to the American Society of Hematology 2011 evidence-based guidelines and the International Consensus Report, Glucocorticoids, IVIg, or Rh immune globulin (RhIg) (anti-D) are recommended as first-line treatments for ITP, and they are used in both children and adults with ITP (1,5,6) (see Table 1). In this review article the focus will be on IVIg therapy used to treat ITP in adult patients.
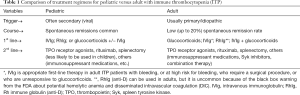
Full table
IVIg efficacy in adults with ITP
IVIg is a blood product enriched with IgG antibodies that is obtained via collection and pooling of human plasma from several thousands of donors (34-38). The manufacturing processes of IVIg include several steps such as precipitation, chromatography techniques and viral inactivation steps to purify the products, maximize tolerability and efficacy, and to minimize side effects (124,125). While the serum IgG is the dominant fraction in all the prepared IVIg, IVIg also contains small amounts of other proteins and components such as albumin, IgA, IgE, IgM, sugars, salts, solvents and buffers, which vary from batch to batch depending on the preparation and manufacturing processes (34,36). These variabilities may contribute to tolerability complications and side effects post infusion (34,36). Therefore, these variables should be carefully considered in relation to the clinical and physiologic conditions of the recipient. In general, IVIg is considered safe and well tolerated with minimal mild and transient side effects (most commonly; headache, fever, chills and nausea) and rare serious adverse events (AEs) such as thrombosis and hemolysis (6,31,36,126) (see below).
IVIg has been used for more than 40 years to treat ITP, initially used to treat primary immunodeficient patients and then later approved to be used to treat several autoimmune diseases and other conditions, including ITP (127-133). In ITP, IVIg is generally considered an effective and safe treatment option as high-quality evidences showed its favorable immunomodulatory effects (134,135). Although the mechanisms of actions of IVIg are complex and still unclear, there are several mechanisms that have been proposed and extrapolated (discussed in further detail below); but most of these mechanisms are extrapolated based on animal models. Nevertheless, in humans, it has been revealed that IVIg plays a role in increasing the platelet lifespan in vivo by reducing the splenic clearance of platelets (136), via Fc-dependent mechanism (6,137).
IVIg was initially shown to be effective in the treatment for ITP when Imbach and colleagues, early in the 1980s, showed an immediate increase in platelet counts in patients with ITP post infusion with IVIg (127). In their study, high-dose IVIg was given at 400 mg/kg over 5 consecutive days. This was the first breakthrough study describing the use of IVIg to treat ITP. Subsequent studies by the same group showed that more than 80% of ITP patients positively responded to IVIg shortly after treatment (138). Later studies confirmed the effectiveness of IVIg in the treatment of ITP in adult populations, with a comparable clinical response rate, leading to the widespread use of IVIg as an immunomodulatory therapy (6,139-141).
As the immunomodulatory effects of IVIg in ITP depend on the administered dose, the efficacy of different IVIg doses was studied to evaluate and establish the optimal IVIg dose for adults with ITP (142,143). As a result, the original/historical dose of IVIg (administrated at 0.4 g/kg daily for 5 days) has been replaced by using a higher initial dose (0.8–1 g/kg) for a short course of treatment, with the possibility to repeat the treatment based on platelet response. A randomized, multicenter trial showed that a 1 g/kg dose of IVIg was more effective in adults with ITP than a 0.5 g/kg regimen, as the results clearly demonstrated a greater increase in platelet count (106×109/L versus 55×109/L) and platelet response rate (67% versus 24%; day 4) in the higher-dose group compared with the lower-dose group (142). In this study, they also showed that additional IVIg doses for a total dose of 2 g/kg for non-responders lead to a response rate of 78% in the entire population in the study. To date, numerous studies and clinical trials have demonstrated that high-dose IVIg (1–2 g/kg) has become an effective first-line therapy in up to 80% of adult patients with ITP (37,40,131,134,144-146). On the basis of these studies, the current dosing guidelines by the American Society of Hematology is administration of 1 g/kg IVIg as an initial single dose, repeated as needed based on platelet response (5).
Noteworthy, while serval studies have demonstrated the effectiveness of IVIg (up to 1 g/kg) in patients with ITP with a response rate around 80%, most of those studies used only 5% IVIg (146-148). Within the past few years, however, higher concentrations of IVIg (10%) products have been developed and introduced as a new therapeutic choice to improve treatment outcomes (17,40). In comparison to 5% IVIg formulation, the 10% IVIg product was associated with shorter infusion time, which resulted in decreasing the patients’ length of stay in hospitals (40,41). Indeed, a number of studies have now been performed to assess the efficacy and safety of several novel human IVIg 10% such as Panzyga® (Octapharma; ready-to-use) (40), 10% IVIG-SN (Green Cross Pharma) (39), Octagam® (Octapharma; ready-to-use) (149) and Privigen® (CSL Behring) (42). Ten percent IVIg is now the standard product used to treat ITP; however, 20% IVIg has recently come onto the market for subcutaneous administration (SCIg) (150-153). Although not currently used to treat ITP, this product shows promise to replace intravenous IVIg administration in the future and further studies will show if this therapeutic approach has efficacy in adult ITP (150). Even though the current IVIg products have a good safety profile and have been shown to be effective treatment for patients with ITP, there are challenges associated with product production, access and availability. Therefore, introduction of new or better products is also needed to maintain a steady and adequate supply and to provide additional options for patients.
While IVIg administered at a dose of 1–2 g/kg typically leads to rapid increases in platelet counts (within 24 to 48 hours) in over 80% of patients, it has been found that the remission post IVIg treatment lasts no longer than 3 to 4 weeks, indicating that this response may be transient (142,154,155). Accordingly, once a patient relapses, particularly when platelet counts fall below 30×109/L, repeat IVIg administration or additional therapy, such as combination therapy using IVIg plus glucocorticoids or second-line therapy such as rituximab, may be necessary as maintenance therapy or to achieve a stable clinical condition for adult patients with ITP (6,139-141,143,155-157). Therefore, a better understanding of the properties and mechanism(s) of action of IVIg, may not only improve the current products but also may influence the future of immunoglobulin-based therapeutics.
IVIg mechanism of action
Following the serendipitous discovery in 1981 by Imbach et al. (127,158) that high-dose IVIg could ameliorate ITP in secondary immune deficiency pediatric patients with concomitant ITP, studies by Fehr et al. (139) showed that following administration of IVIg, clearance of radiolabeled antibody-opsonized red blood cells was inhibited. These observations led to the hypothesis that the mechanism of action of IVIg to ameliorate ITP was through blockade of the Fc receptors (139,159-161) (Figure 1). This amazing discovery of the immunomodulation of ITP by high-dose IVIg caused other clinicians to begin to examine the use of IVIg for the treatment of other autoimmune and inflammatory diseases (162). Indeed, both evidence-based and off-label use of IVIg in many studies demonstrated the utility of using IVIg for the treatment of a variety of conditions (163,164). These clinical findings of the broad immunomodulatory properties of IVIg resulted in the expansion of the possible mechanism(s) of action of this biologic to include immunomodulation due to the anti-inflammatory properties of IVIg (165,166).
As scientists became more interested in how IVIg could ameliorate various diseases, animal models were developed (167). The first studies in animal models were in mice given ITP by passive anti-platelet antibody administration (165,167,168). Studies by different investigators in mouse models of ITP and arthritis revealed a number of possible mechanisms (165,166,168-170). One theory developed over time has been repeatedly propagated (171). In this theory, Ravetch and his protégé Nimmerjahn (165,166,171), have proposed that a small fraction (10%) of total IVIg sialylated in the Fcγ domain, engages the cluster of differentiation 209 (CD209) receptor, also known as DC-SIGN on dendritic cells (172). Engagement of DC-SIGN causes dendritic cells to release interleukin-33 (IL-33) (173). IL-33 is a Th2 polarizing cytokine that would then promote the release of IL-4 from basophils at sites of inflammation. IL-4 acts on macrophages to upregulate the expression of the inhibitory Fcγ receptor (FcγRIIB), which lowers inflammation (174). This model proposes that the subsequent upregulation of FcγRIIB on effector cells raises their threshold for activation, thus allowing for a reduction in phagocytosis of antibody-opsonized platelets and inflammation. Although this model of IVIg action has been touted through numerous publications, it has yet to be supported by other investigators; instead, this model has been refuted by many investigators.
First, passive antibody-induced ITP in mice does not create an inflammatory environment (175) and sialylation of IVIg or a role for FcγRIIB, IL-33, IL-4 or basophils has not been found by other investigators as playing a role in the mechanism of action of IVIg (176-184). However, blockade of FcγR, especially FcγRI and FcγRIII, remains a viable mechanism in ITP. Indeed, studies in humans using an anti-FcγRIII, clone 3G8 or a humanized, deglycosylated variant of 3G8, GMA161, showed improvement in platelet numbers in treatment-refractory ITP patients; however, this amelioration of the ITP was transient (185-187). Recently, it was shown that antibody-coated platelets are removed by macrophages through FcγRI and FcγRIII suggesting that the mechanism of IVIg may be through blockade of both of these receptors (188). Interesting, however, is that it has been shown that it takes about 70–100 times more IVIg than mouse IgG to block in vitro phagocytosis of sheep red blood cell (SRBC)/anti-SRBC using mouse monocytes or RAW 264.7 mouse macrophages (189). These findings raise additional questions as to how IVIg can efficiently ameliorate ITP solely through FcγR blockade.
Earlier findings in the mouse ITP model have shown that IVIg can induce thrombopoiesis as part of its mechanism of action (190). IVIg inducing thrombopoiesis has been found in humans with ITP and is likely part of the amelioration process (191,192); although, the mechanism of how IVIg can induce thrombopoiesis is uncertain. Megakaryopoiesis is regulated by TPO and interleukin-11 (IL-11) (193). Recent work has shown that IVIg can induce IL-11 in mice and in humans (170,175,194). Recombinant human IL-11 (rhuIL-11) is also used to ameliorate chemotherapy-induced thrombocytopenia where it is treatment of choice (195,196). An initial pilot study of using rhuIL-11 to treat 6 patients with treatment-refractory ITP failed to show any efficacy and was moderately toxic (197). However, recent studies are suggesting that use of rhuIL-11 for the treatment of ITP may be helpful (198,199) and appears promising. Indeed, in a mouse model of ITP, it was shown that inhibition of IL-11 using a neutralizing antibody partially prevented the IVIg-mediated amelioration of the ITP. Also, when using recombinant IL-11 instead of IVIg, partial amelioration of the ITP was achieved. Thus, the mechanism of IVIg in amelioration of ITP may involve induction of IL-11 which then acts on megakaryocytes to increase thrombopoiesis (183). This possibility needs additional exploration.
To summarize, the mechanism as to how IVIg ameliorates ITP remains uncertain and because IVIg also ameliorates a number of autoimmune, inflammatory and neuropathy conditions, its mechanism of action is likely complex and involves multiple mechanisms (169,200-202).
Therapies for adult ITP
Therapies for the treatment of adult ITP have advanced considerably over the years (203-208). Today, there are a number of first- and second-line therapies available as well as a number of potential therapeutics in various stages of development. IVIg has become a first-line therapy for ITP in adults. It is of interest to have an overview of ITP first-line therapies and understand how IVIg has become one of the main therapeutics for treatment of this condition. Below, we will explore the history of first-line therapy for ITP.
First-line therapy for ITP
Splenectomy
Historically, the standard first-line therapy for ITP was splenectomy, which is very effective and relatively inexpensive (203,204). Splenectomy for ITP was first performed by Kaznelson in 1916 (203). It has a good track record in the treatment of ITP and was shown to have an approximate 80% response rate, inducing complete remission in approximately 50–70% of patients (204-211); although, relapse of ITP can occur years after the procedure (212). Splenectomy, however, requires surgery under anesthesia and, therefore, has the possibility of severe complications due to the surgery (212-216). With the advent of therapies having fewer potential complications compared to splenectomy, such as immune suppressants, IVIg and RhIg anti-D therapies, splenectomy was relegated to a last-resort second-line therapy. Today it is recommended to only be used in adults with ITP for ≥3 months who are glucocorticoid-dependent or have no response to these steroids (9).
Glucocorticoids
Commonly referred to as “steroids”, corticosteroids comprise two groups of adrenal cortex-produced steroids, some of which are used clinically. Glucocorticoids (GCs) represent the most important and frequently used class of drugs in the management of many inflammatory and immunologic conditions (217). The glucocorticoid prednisone was first used to treat ITP in 1958 (218). Because of its efficacy for amelioration of ITP (82) and less significant side effects compared to splenectomy, prednisone became a first-line therapy for ITP in 1982 in Japan and in 1996 in the USA (82). Prednisone and dexamethasone are the glucocorticoids of choice for first-line therapy of ITP (9,82,219-221). GCs are known to enter many different cell types through a receptor-mediated mechanism or passage directly into the cell. Once inside the cell cytosol, GCs bind to glucocorticoid receptors. This interaction results in significant effects on signal transduction, which in turn affects the normal function of cells. The final result is a profound effect of glucocorticoids on the immune response and immune cell function, such as macrophage phagocytosis and antibody production (222) (Figure 1B). Unfortunately, glucocorticoids are also associated with serious side effects. Adrenal suppression, dyslipidemia, hyperglycemia, Cushing’s syndrome, cardiovascular disease, osteoporosis, psychiatric disturbances, and immunosuppression are among the most important side effects of glucocorticoids (223). These side effects are especially noticeable at high doses for prolonged periods. Despite these drawbacks, glucocorticoid therapy remains a first-line therapeutic approach to the resolution of ITP. However, there remains cases of resistance to glucocorticoids; therefore, other additions to the first-line of therapy were needed and this was solved by the serendipitous discovery that immunoglobulin therapy was highly efficacious at amelioration of ITP (158,224) (Figure 1C).
IVIg and anti-D
The use of IVIg for the treatment of ITP was first described in 1981 by Imbach et al. (127,158). High-doses of IVIg (1 to 2 g/kg) were first used in a pediatric patient having secondary immune deficiency complicated with ITP (127,158). Surprisingly, this patient and the subsequent patients, showed an increase in platelet numbers following the IVIg therapy (139,159,225,226). Later studies used high-dose IVIg therapy in adult ITP with some mixed results but, generally, it worked and, along with glucocorticoids and anti-D, IVIg is now a standard first-line therapy for adult ITP (205-212,226) (see below about anti-D and combination therapy).
Although IVIg has been shown to be well tolerated with few and mild side effects, with headache the primary complaint, it does, nevertheless cause some rare but significant AEs that include thrombosis (227,228) and hemolysis due to the anti-A and anti-B iso-agglutinins contained in the product (229). IVIg-associated hemolysis is more common that thought and can result in life-threatening hemolysis following high-dose therapy (229,230).
Of interest is the fact that early IVIg products contained various alloantibodies to red blood cells that were not iso-agglutinins. Antibodies such as anti-Rhesus (RhD), anti-D, were rather common contaminants of IVIg (231,232). These antibodies, especially anti-D, were thought to be responsible for the low to modest hemolysis that was routinely seen when using IVIg to treat ITP patients (233). This antibody-induced hemolysis was perceived by Mueller-Eckhart’s team to be mostly due to anti-D contained in the IVIg product and led these investigators to try anti-D itself as a therapy for ITP (233). Their thinking was that the mechanism of IVIg amelioration of ITP may involve an antibody-RBC interaction that results in hemolysis. These investigators were the first to use RhIg containing high levels of anti-D to treat ITP patients; and it worked (233-235). Thus, use of RhIg (anti-D) became a first-line of therapy for ITP patients (9). One limitation of this approach was, as indicated above that it only was efficacious in Rh(D)-positive patients. However, a larger limitation was the fact that in some patients treated with anti-D that were Rh(D)-positive, the anti-D resulted in significant morbidity due to associated problems resulting from the hemolysis, forcing the food and drug administration to issue a “black box warning” for using anti-D to treat ITP, especially in pediatric patients (236-239). Anti-D is, nevertheless, still used in the treatment of both pediatric and adult ITP (9,206-212). However, the AEs associated with IVIg, anti-D and glucocorticoids have prompted investigators to explore replacement of IVIg and other alternatives for the treatment of ITP.
Combination therapy
In cases where first-line therapy with IVIg is unsuccessful, a combination of IVIg with glucocorticoids is often used (157). In one study, it was found that use of IVIg plus glucocorticoids was more efficacious than glucocorticoids alone having significantly higher response rates (240). In another case, use of IVIg plus dexamethasone was found to be effective at resulting in a favorable outcome in a patient having coronavirus disease 2019 (COVID-19) and severe ITP (241). When patients are refractory to first-line therapeutics or combinations, splenectomy is still practiced as a “last resort” in some adult patients, especially if also refractory to second-line therapeutics.
Summary
IVIg is a biologic manufactured from tens of thousands of human plasma donations and had become a first-line treatment modality for amelioration of ITP. It has been used for more than 40 years in both pediatrics and adult patients. In adult ITP, IVIg 10% had often been the treatment of choice over glucocorticoids due to the lessor AEs associated with IVIg therapy. Although anti-D (RhIg) therapy continues to be used, the much harder to produce product from a small population of Rh-negative donors as well as the significant AEs associated with its use have enhanced the use of glucocorticoids and IVIg in the treatment of ITP over the years.
Recently, treatment guidelines for adults with ITP have evolved to favor glucocorticoids over IVIg for first-line therapy. This is primarily due to the less cost of glucocorticoids compared to IVIg and the fact that, in adults with ITP, glucocorticoids are very effective first-line therapy (208,209). However, IVIg and RhIg continue to be used by some institutions as first-line treatment and, especially, where patients may be bleeding (10,208,209). Although some recommendations continue to include IVIg and RhIg in their approach to adult ITP (208,209), more consensus is emerging that IVIg is best saved for adults who have active bleeding or at high risk for bleeding, who require a surgical procedure, or those adults who are unresponsive to glucocorticoids (10). In recent recommendations from the American Society for Hematology, IVIg is not included in first-line therapy (9,25). Thus, in many situations due to recent recommendations, IVIg has been relegated to second-line therapy for adult ITP (see Table 1 for a comparison of therapy for pediatrics versus adult ITP patients).
Acknowledgments
Funding: Dr. Ruqayyah J. Almizraq is supported by a Post-doctoral Fellowship from the Canadian Blood Services.
Footnote
Provenance and Peer Review: This article was commissioned by the Guest Editors (John W. Semple and Rick Kapur) for the series “Treatment of Immune Thrombocytopenia (ITP)” published in Annals of Blood. The article was sent for external peer review organized by the Guest Editors and the editorial office.
Conflicts of Interest: Both authors have completed the ICMJE uniform disclosure form (available at http://dx.doi.org/10.21037/aob-20-87). The series “Treatment of Immune Thrombocytopenia (ITP)” was commissioned by the editorial office without any funding or sponsorship. Dr. DRB reports other from CSL Behring Ltd., outside the submitted work. RJA has no other conflicts of interest to declare.
Ethical Statement: The authors are accountable for all aspects of the work in ensuring that questions related to the accuracy or integrity of any part of the work are appropriately investigated and resolved.
Open Access Statement: This is an Open Access article distributed in accordance with the Creative Commons Attribution-NonCommercial-NoDerivs 4.0 International License (CC BY-NC-ND 4.0), which permits the non-commercial replication and distribution of the article with the strict proviso that no changes or edits are made and the original work is properly cited (including links to both the formal publication through the relevant DOI and the license). See: https://creativecommons.org/licenses/by-nc-nd/4.0/.
References
- Rodeghiero F, Ruggeri M. ITP and international guidelines: what do we know, what do we need? Presse Med 2014;43:e61-7. [Crossref] [PubMed]
- Rodeghiero F, Stasi R, Gernsheimer T, et al. Standardization of terminology, definitions and outcome criteria in immune thrombocytopenic purpura of adults and children: report from an international working group. Blood 2009;113:2386-93. [Crossref] [PubMed]
- Terrell DR, Beebe LA, Vesely SK, et al. The incidence of immune thrombocytopenic purpura in children and adults: A critical review of published reports. Am J Hematol 2010;85:174-80. [Crossref] [PubMed]
- Moulis G, Palmaro A, Montastruc JL, et al. Epidemiology of incident immune thrombocytopenia: a nationwide population-based study in France. Blood 2014;124:3308-15. [Crossref] [PubMed]
- Neunert C, Lim W, Crowther M, et al. The American Society of Hematology 2011 evidence-based practice guideline for immune thrombocytopenia. Blood 2011;117:4190-207. [Crossref] [PubMed]
- Provan D, Stasi R, Newland AC, et al. International consensus report on the investigation and management of primary immune thrombocytopenia. Blood 2010;115:168-86. [Crossref] [PubMed]
- Provan D, Newland AC. Current Management of Primary Immune Thrombocytopenia. Adv Ther 2015;32:875-87. [Crossref] [PubMed]
- Lambert MP, Gernsheimer TB. Clinical updates in adult immune thrombocytopenia. Blood 2017;129:2829-35. [Crossref] [PubMed]
- Neunert C, Terrell DR, Arnold DM, et al. American Society of Hematology 2019 guidelines for immune thrombocytopenia. Blood Adv 2019;3:3829-66. [Crossref] [PubMed]
- Provan D, Arnold DM, Bussel JB, et al. Updated international consensus report on the investigation and management of primary immune thrombocytopenia. Blood Adv 2019;3:3780-817. [Crossref] [PubMed]
- Neunert C, Noroozi N, Norman G, et al. Severe bleeding events in adults and children with primary immune thrombocytopenia: a systematic review. J Thromb Haemost 2015;13:457-64. [Crossref] [PubMed]
- Rodeghiero F, Michel M, Gernsheimer T, et al. Standardization of bleeding assessment in immune thrombocytopenia: report from the International Working Group. Blood 2013;121:2596-606. [Crossref] [PubMed]
- Psaila B, Petrovic A, Page LK, et al. Intracranial hemorrhage (ICH) in children with immune thrombocytopenia (ITP): study of 40 cases. Blood 2009;114:4777-83. [Crossref] [PubMed]
- Altomare I, Cetin K, Wetten S, et al. Rate of bleeding-related episodes in adult patients with primary immune thrombocytopenia: a retrospective cohort study using a large administrative medical claims database in the US. Clin Epidemiol 2016;8:231-9. [PubMed]
- Middelburg RA, Carbaat-Ham JC, Hesam H, et al. Platelet function in adult ITP patients can be either increased or decreased, compared to healthy controls, and is associated with bleeding risk. Hematology 2016;21:549-51. [Crossref] [PubMed]
- Panzer S, Rieger M, Vormittag R, et al. Platelet function to estimate the bleeding risk in autoimmune thrombocytopenia. Eur J Clin Invest 2007;37:814-9. [Crossref] [PubMed]
- Witkowski M, Witkowska M, Robak T. Autoimmune thrombocytopenia: Current treatment options in adults with a focus on novel drugs. Eur J Haematol 2019;103:531-41. [Crossref] [PubMed]
- Kühne T, Imbach P, Bolton-Maggs PH, et al. Newly diagnosed idiopathic thrombocytopenic purpura in childhood: an observational study. Lancet 2001;358:2122-5. [Crossref] [PubMed]
- Zeller B, Rajantie J, Hedlund-Treutiger I, et al. Childhood idiopathic thrombocytopenic purpura in the Nordic countries: epidemiology and predictors of chronic disease. Acta Paediatr 2005;94:178-84. [Crossref] [PubMed]
- Despotovic JM, Grimes AB. Pediatric ITP: is it different from adult ITP? Hematology Am Soc Hematol Educ Program 2018;2018:405-11. [Crossref] [PubMed]
- Despotovic JM, Lambert MP, Herman JH, et al. RhIG for the treatment of immune thrombocytopenia: consensus and controversy (CME). Transfusion 2012;52:1126-36; quiz 5. [Crossref] [PubMed]
- Tinazzi E, Osti N, Beri R, et al. Pathogenesis of immune thrombocytopenia in common variable immunodeficiency. Autoimmun Rev 2020;19:102616 [Crossref] [PubMed]
- George JN, Woolf SH, Raskob GE, et al. Idiopathic thrombocytopenic purpura: a practice guideline developed by explicit methods for the American Society of Hematology. Blood 1996;88:3-40. [Crossref] [PubMed]
- British Committee for Standards in Haematology General Haematology Task Force. Guidelines for the investigation and management of idiopathic thrombocytopenic purpura in adults, children and in pregnancy. Br J Haematol 2003;120:574-96. [Crossref] [PubMed]
- Neunert CE, Cooper N. Evidence-based management of immune thrombocytopenia: ASH guideline update. Hematology Am Soc Hematol Educ Program 2018;2018:568-75. [Crossref] [PubMed]
- Cuker A, Cines DB, Neunert CE. Controversies in the treatment of immune thrombocytopenia. Curr Opin Hematol 2016;23:479-85. [Crossref] [PubMed]
- Matschke J, Muller-Beissenhirtz H, Novotny J, et al. A Randomized Trial of Daily Prednisone versus Pulsed Dexamethasone in Treatment-Naive Adult Patients with Immune Thrombocytopenia: EIS 2002 Study. Acta Haematol 2016;136:101-7. [Crossref] [PubMed]
- Wei Y, Ji XB, Wang YW, et al. High-dose dexamethasone vs prednisone for treatment of adult immune thrombocytopenia: a prospective multicenter randomized trial. Blood 2016;127:296-302; quiz 70. [Crossref] [PubMed]
- Neunert CE. Management of newly diagnosed immune thrombocytopenia: can we change outcomes? Hematology Am Soc Hematol Educ Program 2017;2017:400-5. [Crossref] [PubMed]
- Branch DR. Anti-A and anti-B: what are they and where do they come from? Transfusion 2015;55:S74-9. [Crossref] [PubMed]
- Branch DR, Hellberg A, Bruggeman CW, et al. ABO zygosity, but not secretor or Fc receptor status, is a significant risk factor for IVIG-associated hemolysis. Blood 2018;131:830-5. [Crossref] [PubMed]
- Godeau B, Chevret S, Varet B, et al. Intravenous immunoglobulin or high-dose methylprednisolone, with or without oral prednisone, for adults with untreated severe autoimmune thrombocytopenic purpura: a randomised, multicentre trial. Lancet 2002;359:23-9. [Crossref] [PubMed]
- Lo E, Deane S. Diagnosis and classification of immune-mediated thrombocytopenia. Autoimmun Rev 2014;13:577-83. [Crossref] [PubMed]
- Prins C, Gelfand EW, French LE. Intravenous immunoglobulin: properties, mode of action and practical use in dermatology. Acta Derm Venereol 2007;87:206-18. [PubMed]
- Bayry J, Negi VS, Kaveri SV. Intravenous immunoglobulin therapy in rheumatic diseases. Nat Rev Rheumatol 2011;7:349-59. [Crossref] [PubMed]
- Gelfand EW. Differences between IGIV products: impact on clinical outcome. Int Immunopharmacol 2006;6:592-9. [Crossref] [PubMed]
- Lazarus AH, Crow AR. Mechanism of action of IVIG and anti-D in ITP. Transfus Apher Sci 2003;28:249-55. [Crossref] [PubMed]
- Sewell WA, Jolles S. Immunomodulatory action of intravenous immunoglobulin. Immunology 2002;107:387-93. [Crossref] [PubMed]
- Hong J, Bang SM, Mun YC, et al. Efficacy and Safety of a New 10% Intravenous Immunoglobulin Product in Patients with Primary Immune Thrombocytopenia (ITP). J Korean Med Sci 2018;33:e142 [Crossref] [PubMed]
- Arbach O, Taumberger AB, Wietek S, et al. Efficacy and safety of a new intravenous immunoglobulin (Panzyga®) in chronic immune thrombocytopenia. Transfus Med 2019;29:48-54. [Crossref] [PubMed]
- Robak T, Mainau C, Pyringer B, et al. Efficacy and safety of a new intravenous immunoglobulin 10% formulation (octagam® 10%) in patients with immune thrombocytopenia. Hematology 2010;15:351-9. [Crossref] [PubMed]
- Robak T, Salama A, Kovaleva L, et al. Efficacy and safety of Privigen, a novel liquid intravenous immunoglobulin formulation, in adolescent and adult patients with chronic immune thrombocytopenic purpura. Hematology 2009;14:227-36. [Crossref] [PubMed]
- Gernsheimer T. Chronic idiopathic thrombocytopenic purpura: mechanisms of pathogenesis. Oncologist 2009;14:12-21. [Crossref] [PubMed]
- Stratton JR, Ballem PJ, Gernsheimer T, et al. Platelet destruction in autoimmune thrombocytopenic purpura: kinetics and clearance of indium-111-labeled autologous platelets. J Nucl Med 1989;30:629-37. [PubMed]
- Cines DB, Cuker A, Semple JW. Pathogenesis of immune thrombocytopenia. Presse Med 2014;43:e49-e59. [Crossref] [PubMed]
- Zufferey A, Kapur R, Semple JW. Pathogenesis and Therapeutic Mechanisms in Immune Thrombocytopenia (ITP). J Clin Med 2017;6:16. [Crossref] [PubMed]
- Harrington WJ, Minnich V, Hollingsworth JW, et al. Demonstration of a thrombocytopenic factor in the blood of patients with thrombocytopenic purpura. J Lab Clin Med 1951;38:1-10. [PubMed]
- Karpatkin S, Siskind GW. In vitro detection of platelet antibody in patients with idiopathic thrombocytopenic purpura and systemic lupus erythematosus. Blood 1969;33:795-812. [Crossref] [PubMed]
- He R, Reid DM, Jones CE, et al. Spectrum of Ig classes, specificities, and titers of serum antiglycoproteins in chronic idiopathic thrombocytopenic purpura. Blood 1994;83:1024-32. [Crossref] [PubMed]
- van Leeuwen EF, van der Ven JT, Engelfriet CP, et al. Specificity of autoantibodies in autoimmune thrombocytopenia. Blood 1982;59:23-6. [Crossref] [PubMed]
- McMillan R. Autoantibodies and autoantigens in chronic immune thrombocytopenic purpura. Semin Hematol 2000;37:239-48. [Crossref] [PubMed]
- Escher R, Muller D, Vogel M, et al. Recombinant human natural autoantibodies against GPIIb/IIIa inhibit binding of autoantibodies from patients with AITP. Br J Haematol 1998;102:820-8. [Crossref] [PubMed]
- Audia S, Mahevas M, Samson M, et al. Pathogenesis of immune thrombocytopenia. Autoimmun Rev 2017;16:620-32. [Crossref] [PubMed]
- Roark JH, Bussel JB, Cines DB, et al. Genetic analysis of autoantibodies in idiopathic thrombocytopenic purpura reveals evidence of clonal expansion and somatic mutation. Blood 2002;100:1388-98. [Crossref] [PubMed]
- Cooper N, Ghanima W. Immune Thrombocytopenia. N Engl J Med 2019;381:945-55. [Crossref] [PubMed]
- McKenzie CG, Guo L, Freedman J, et al. Cellular immune dysfunction in immune thrombocytopenia (ITP). Br J Haematol 2013;163:10-23. [Crossref] [PubMed]
- Lazarus AH, Semple JW, Cines DB. Innate and adaptive immunity in immune thrombocytopenia. Semin Hematol 2013;50:S68-70. [Crossref] [PubMed]
- Rogier T, Samson M, Mourey G, et al. Antiplatelet Antibodies Do Not Predict the Response to Intravenous Immunoglobulins during Immune Thrombocytopenia. J Clin Med 2020;9:1998. [Crossref] [PubMed]
- Li J, Sullivan JA, Ni H. Pathophysiology of immune thrombocytopenia. Curr Opin Hematol 2018;25:373-81. [Crossref] [PubMed]
- Grozovsky R, Begonja AJ, Liu K, et al. The Ashwell-Morell receptor regulates hepatic thrombopoietin production via JAK2-STAT3 signaling. Nat Med 2015;21:47-54. [Crossref] [PubMed]
- Qiu J, Liu X, Li X, et al. CD8(+) T cells induce platelet clearance in the liver via platelet desialylation in immune thrombocytopenia. Sci Rep 2016;6:27445. [Crossref] [PubMed]
- Perera M, Garrido T. Advances in the pathophysiology of primary immune thrombocytopenia. Hematology 2017;22:41-53. [Crossref] [PubMed]
- Breunis WB, van Mirre E, Bruin M, et al. Copy number variation of the activating FCGR2C gene predisposes to idiopathic thrombocytopenic purpura. Blood 2008;111:1029-38. [Crossref] [PubMed]
- Carcao MD, Blanchette VS, Wakefield CD, et al. Fcgamma receptor IIa and IIIa polymorphisms in childhood immune thrombocytopenic purpura. Br J Haematol 2003;120:135-41. [Crossref] [PubMed]
- Foster CB, Zhu S, Erichsen HC, et al. Polymorphisms in inflammatory cytokines and Fcgamma receptors in childhood chronic immune thrombocytopenic purpura: a pilot study. Br J Haematol 2001;113:596-9. [Crossref] [PubMed]
- Pehlivan M, Okan V, Sever T, et al. Investigation of TNF-alpha, TGF-beta 1, IL-10, IL-6, IFN-gamma, MBL, GPIA, and IL1A gene polymorphisms in patients with idiopathic thrombocytopenic purpura. Platelets 2011;22:588-95. [Crossref] [PubMed]
- Rischewski JR, Imbach P, Paulussen M, et al. Idiopathic thrombocytopenic purpura (ITP): is there a genetic predisposition? Pediatr Blood Cancer 2006;47:678-80. [Crossref] [PubMed]
- Wu KH, Peng CT, Li TC, et al. Interleukin 4, interleukin 6 and interleukin 10 polymorphisms in children with acute and chronic immune thrombocytopenic purpura. Br J Haematol 2005;128:849-52. [Crossref] [PubMed]
- Li Z, Nardi MA, Karpatkin S. Role of molecular mimicry to HIV-1 peptides in HIV-1-related immunologic thrombocytopenia. Blood 2005;106:572-6. [Crossref] [PubMed]
- Rand ML, Wright JF. Virus-associated idiopathic thrombocytopenic purpura. Transfus Sci 1998;19:253-9. [Crossref] [PubMed]
- Takahashi T, Yujiri T, Shinohara K, et al. Molecular mimicry by Helicobacter pylori CagA protein may be involved in the pathogenesis of H. pylori-associated chronic idiopathic thrombocytopenic purpura. Br J Haematol 2004;124:91-6. [Crossref] [PubMed]
- Zhang W, Nardi MA, Borkowsky W, et al. Role of molecular mimicry of hepatitis C virus protein with platelet GPIIIa in hepatitis C-related immunologic thrombocytopenia. Blood 2009;113:4086-93. [Crossref] [PubMed]
- Stasi R, Sarpatwari A, Segal JB, et al. Effects of eradication of Helicobacter pylori infection in patients with immune thrombocytopenic purpura: a systematic review. Blood 2009;113:1231-40. [Crossref] [PubMed]
- McMillan R, Wang L, Tomer A, et al. Suppression of in vitro megakaryocyte production by antiplatelet autoantibodies from adult patients with chronic ITP. Blood 2004;103:1364-9. [Crossref] [PubMed]
- Najaoui A, Bakchoul T, Stoy J, et al. Autoantibody-mediated complement activation on platelets is a common finding in patients with immune thrombocytopenic purpura (ITP). Eur J Haematol 2012;88:167-74. [Crossref] [PubMed]
- Stasi R, Cooper N, Del Poeta G, et al. Analysis of regulatory T-cell changes in patients with idiopathic thrombocytopenic purpura receiving B cell-depleting therapy with rituximab. Blood 2008;112:1147-50. [Crossref] [PubMed]
- Hauch TW, Rosse WF. Platelet-bound complement (C3) in immune thrombocytopenia. Blood 1977;50:1129-36. [Crossref] [PubMed]
- Tsubakio T, Tani P, Curd JG, et al. Complement activation in vitro by antiplatelet antibodies in chronic immune thrombocytopenic purpura. Br J Haematol 1986;63:293-300. [Crossref] [PubMed]
- Harker LA. Thrombokinetics in idiopathic thrombocytopenic purpura. Br J Haematol 1970;19:95-104. [Crossref] [PubMed]
- Harker LA, Finch CA. Thrombokinetics in man. J Clin Invest 1969;48:963-74. [Crossref] [PubMed]
- Branehög I, Kutti J, Ridell B, et al. The relation of thrombokinetics to bone marrow megakaryocytes in idiopathic thrombocytopenic purpura (ITP). Blood 1975;45:551-62. [Crossref] [PubMed]
- Branehög I, Kutti J, Weinfeld A. Platelet survival and platelet production in idiopathic thrombocytopenic purpura (ITP). Br J Haematol 1974;27:127-43. [Crossref] [PubMed]
- Kernoff LM, Blake KC, Shackleton D. Influence of the amount of platelet-bound IgG on platelet survival and site of sequestration in autoimmune thrombocytopenia. Blood 1980;55:730-3. [Crossref] [PubMed]
- Blanchette M, Freedman J. The history of idiopathic thrombocytopenic purpura (ITP). Transfus Sci 1998;19:231-6. [Crossref] [PubMed]
- Louwes H, Vellenga E, Houwerzijl EJ, et al. Effects of prednisone and splenectomy in patients with idiopathic thrombocytopenic purpura: only splenectomy induces a complete remission. Ann Hematol 2001;80:728-32. [Crossref] [PubMed]
- Nugent D, McMillan R, Nichol JL, et al. Pathogenesis of chronic immune thrombocytopenia: increased platelet destruction and/or decreased platelet production. Br J Haematol 2009;146:585-96. [Crossref] [PubMed]
- Khodadi E, Asnafi AA, Shahrabi S, et al. Bone marrow niche in immune thrombocytopenia: a focus on megakaryopoiesis. Ann Hematol 2016;95:1765-76. [Crossref] [PubMed]
- Dameshek W, Miller EB. The megakaryocytes in idiopathic thrombocytopenic purpura, a form of hypersplenism. Blood 1946;1:27-50. [Crossref] [PubMed]
- Pisciotta AV, Stefanini M, Dameshek W. Studies on platelets. X. Morphologic characteristics of megakaryocytes by phase contrast microscopy in normals and in patients with idiopathic thrombocytopenic purpura. Blood 1953;8:703-23. [Crossref] [PubMed]
- Bakchoul T, Sachs UJ. Platelet destruction in immune thrombocytopenia. Understanding the mechanisms. Hamostaseologie 2016;36:187-94. [PubMed]
- Malara A, Abbonante V, Di Buduo CA, et al. The secret life of a megakaryocyte: emerging roles in bone marrow homeostasis control. Cell Mol Life Sci 2015;72:1517-36. [Crossref] [PubMed]
- Iraqi M, Perdomo J, Yan F, et al. Immune thrombocytopenia: antiplatelet autoantibodies inhibit proplatelet formation by megakaryocytes and impair platelet production in vitro. Haematologica 2015;100:623-32. [Crossref] [PubMed]
- Li S, Wang L, Zhao C, et al. CD8+ T cells suppress autologous megakaryocyte apoptosis in idiopathic thrombocytopenic purpura. Br J Haematol 2007;139:605-11. [Crossref] [PubMed]
- Olsson B, Ridell B, Carlsson L, et al. Recruitment of T cells into bone marrow of ITP patients possibly due to elevated expression of VLA-4 and CX3CR1. Blood 2008;112:1078-84. [Crossref] [PubMed]
- Kuefer MU, Wang WC, Head DR, et al. Thrombopoietin level in young patients is related to megakaryocyte frequency and platelet count. J Pediatr Hematol Oncol 1998;20:36-43. [Crossref] [PubMed]
- Emmons RV, Reid DM, Cohen RL, et al. Human thrombopoietin levels are high when thrombocytopenia is due to megakaryocyte deficiency and low when due to increased platelet destruction. Blood 1996;87:4068-71. [Crossref] [PubMed]
- de Sauvage FJ, Carver-Moore K, Luoh SM, et al. Physiological regulation of early and late stages of megakaryocytopoiesis by thrombopoietin. J Exp Med 1996;183:651-6. [Crossref] [PubMed]
- Kaushansky K. The molecular mechanisms that control thrombopoiesis. J Clin Invest 2005;115:3339-47. [Crossref] [PubMed]
- Kuter DJ, Beeler DL, Rosenberg RD. The purification of megapoietin: a physiological regulator of megakaryocyte growth and platelet production. Proc Natl Acad Sci U S A 1994;91:11104-8. [Crossref] [PubMed]
- Nichol JL, Hokom MM, Hornkohl A, et al. Megakaryocyte growth and development factor. Analyses of in vitro effects on human megakaryopoiesis and endogenous serum levels during chemotherapy-induced thrombocytopenia. J Clin Invest 1995;95:2973-8. [Crossref] [PubMed]
- Usuki K, Tahara T, Iki S, et al. Serum thrombopoietin level in various hematological diseases. Stem Cells 1996;14:558-65. [Crossref] [PubMed]
- Marsh JC, Gibson FM, Prue RL, et al. Serum thrombopoietin levels in patients with aplastic anaemia. Br J Haematol 1996;95:605-10. [Crossref] [PubMed]
- Aledort LM, Hayward CP, Chen MG, et al. Prospective screening of 205 patients with ITP, including diagnosis, serological markers, and the relationship between platelet counts, endogenous thrombopoietin, and circulating antithrombopoietin antibodies. Am J Hematol 2004;76:205-13. [Crossref] [PubMed]
- Chang M, Suen Y, Meng G, et al. Differential mechanisms in the regulation of endogenous levels of thrombopoietin and interleukin-11 during thrombocytopenia: insight into the regulation of platelet production. Blood 1996;88:3354-62. [Crossref] [PubMed]
- Ichikawa N, Ishida F, Shimodaira S, et al. Regulation of serum thrombopoietin levels by platelets and megakaryocytes in patients with aplastic anaemia and idiopathic thrombocytopenic purpura. Thromb Haemost 1996;76:156-60. [Crossref] [PubMed]
- Kosugi S, Kurata Y, Tomiyama Y, et al. Circulating thrombopoietin level in chronic immune thrombocytopenic purpura. Br J Haematol 1996;93:704-6. [Crossref] [PubMed]
- Houwerzijl EJ, Blom NR, van der Want JJ, et al. Megakaryocytic dysfunction in myelodysplastic syndromes and idiopathic thrombocytopenic purpura is in part due to different forms of cell death. Leukemia 2006;20:1937-42. [Crossref] [PubMed]
- Houwerzijl EJ, Blom NR, van der Want JJ, et al. Ultrastructural study shows morphologic features of apoptosis and para-apoptosis in megakaryocytes from patients with idiopathic thrombocytopenic purpura. Blood 2004;103:500-6. [Crossref] [PubMed]
- Swinkels M, Rijkers M, Voorberg J, et al. Emerging Concepts in Immune Thrombocytopenia. Front Immunol 2018;9:880. [Crossref] [PubMed]
- Schulze H, Gaedicke G. Immune thrombocytopenia in children and adults: what's the same, what's different? Haematologica 2011;96:1739-41. [Crossref] [PubMed]
- Bennett CM, Neunert C, Grace RF, et al. Predictors of remission in children with newly diagnosed immune thrombocytopenia: Data from the Intercontinental Cooperative ITP Study Group Registry II participants. Pediatr Blood Cancer 2018;65. [Crossref] [PubMed]
- Segal JB, Powe NR. Prevalence of immune thrombocytopenia: analyses of administrative data. J Thromb Haemost 2006;4:2377-83. [Crossref] [PubMed]
- Neylon AJ, Saunders PW, Howard MR, et al. Clinically significant newly presenting autoimmune thrombocytopenic purpura in adults: a prospective study of a population-based cohort of 245 patients. Br J Haematol 2003;122:966-74. [Crossref] [PubMed]
- Terrell DR, Beebe LA, Neas BR, et al. Prevalence of primary immune thrombocytopenia in Oklahoma. Am J Hematol 2012;87:848-52. [Crossref] [PubMed]
- Bennett D, Hodgson ME, Shukla A, et al. Prevalence of diagnosed adult immune thrombocytopenia in the United Kingdom. Adv Ther 2011;28:1096-104. [Crossref] [PubMed]
- Fogarty PF. Chronic immune thrombocytopenia in adults: epidemiology and clinical presentation. Hematol Oncol Clin North Am 2009;23:1213-21. [Crossref] [PubMed]
- Schifferli A, Holbro A, Chitlur M, et al. A comparative prospective observational study of children and adults with immune thrombocytopenia: 2-year follow-up. Am J Hematol 2018;93:751-9. [Crossref] [PubMed]
- Cohen YC, Djulbegovic B, Shamai-Lubovitz O, et al. The bleeding risk and natural history of idiopathic thrombocytopenic purpura in patients with persistent low platelet counts. Arch Intern Med 2000;160:1630-8. [Crossref] [PubMed]
- Nampoothiri RV, Singh C, Lad D, et al. Immune Thrombocytopenia is Still the Commonest Diagnosis on Consultative Hematology. Indian J Hematol Blood Transfus 2019;35:352-6. [Crossref] [PubMed]
- Arnold DM, Kelton JG. Current options for the treatment of idiopathic thrombocytopenic purpura. Semin Hematol 2007;44:S12-23. [Crossref] [PubMed]
- Hicks LK, Bering H, Carson KR, et al. Five hematologic tests and treatments to question. Hematology Am Soc Hematol Educ Program 2014;2014:599-603. [Crossref] [PubMed]
- Grainger JD, Rees JL, Reeves M, et al. Changing trends in the UK management of childhood ITP. Arch Dis Child 2012;97:8-11. [Crossref] [PubMed]
- Schultz CL, Mitra N, Schapira MM, et al. Influence of the American Society of Hematology guidelines on the management of newly diagnosed childhood immune thrombocytopenia. JAMA Pediatr 2014;168:e142214 [Crossref] [PubMed]
- Radosevich M, Burnouf T. Intravenous immunoglobulin G: trends in production methods, quality control and quality assurance. Vox Sang 2010;98:12-28. [Crossref] [PubMed]
- Martin TD. IGIV: contents, properties, and methods of industrial production--evolving closer to a more physiologic product. Int Immunopharmacol 2006;6:517-22. [Crossref] [PubMed]
- Jandus C, Simon H-U, von Gunten S. Targeting siglecs--a novel pharmacological strategy for immuno- and glycotherapy. Biochem Pharmacol 2011;82:323-32. [Crossref] [PubMed]
- Imbach P, Barandun S, Baumgartner C, et al. High-dose intravenous gammaglobulin therapy of refractory, in particular idiopathic thrombocytopenia in childhood. Helv Paediatr Acta 1981;36:81-6. [PubMed]
- Bruton OC. Agammaglobulinemia. Pediatrics 1952;9:722-8. [PubMed]
- Barahona Afonso AF, Joao CM. The Production Processes and Biological Effects of Intravenous Immunoglobulin. Biomolecules 2016;6:15. [Crossref] [PubMed]
- Perez EE, Orange JS, Bonilla F, et al. Update on the use of immunoglobulin in human disease: A review of evidence. J Allergy Clin Immunol 2017;139:S1-S46. [Crossref] [PubMed]
- Etzioni A, Pollack S. High dose intravenous gammaglobulins in autoimmune disorders: mode of action and therapeutic uses. Autoimmunity 1989;3:307-15. [Crossref] [PubMed]
- Stangel M, Hartung HP, Marx P, et al. Intravenous immunoglobulin treatment of neurological autoimmune diseases. J Neurol Sci 1998;153:203-14. [Crossref] [PubMed]
- Jolles S, Chapel H, Litzman J. When to initiate immunoglobulin replacement therapy (IGRT) in antibody deficiency: a practical approach. Clin Exp Immunol 2017;188:333-41. [Crossref] [PubMed]
- Bierling P, Godeau B. Intravenous immunoglobulin and autoimmune thrombocytopenic purpura: 22 years on. Vox Sang 2004;86:8-14. [Crossref] [PubMed]
- Liu J, Pavenski K, Sholzberg M. Appropriateness of intravenous immunoglobulin use in immune thrombocytopenia (ITP): A Canadian centre deep dive audit. Transfus Apher Sci 2019;58:491-4. [Crossref] [PubMed]
- Newland AC, Macey MG. Immune thrombocytopenia and Fc receptor-mediated phagocyte function. Ann Hematol 1994;69:61-7. [Crossref] [PubMed]
- Cines DB, Bussel JB, Liebman HA, et al. The ITP syndrome: pathogenic and clinical diversity. Blood 2009;113:6511-21. [Crossref] [PubMed]
- Imbach P, Wagner HP, Berchtold W, et al. Intravenous immunoglobulin versus oral corticosteroids in acute immune thrombocytopenic purpura in childhood. Lancet 1985;2:464-8. [Crossref] [PubMed]
- Fehr J, Hofmann V, Kappeler U. Transient reversal of thrombocytopenia in idiopathic thrombocytopenic purpura by high-dose intravenous gamma globulin. N Engl J Med 1982;306:1254-8. [Crossref] [PubMed]
- Bussel JB, Kimberly RP, Inman RD, et al. Intravenous gammaglobulin treatment of chronic idiopathic thrombocytopenic purpura. Blood 1983;62:480-6. [Crossref] [PubMed]
- Newland AC, Treleaven JG, Minchinton RM, et al. High-dose intravenous IgG in adults with autoimmune thrombocytopenia. Lancet 1983;1:84-7. [Crossref] [PubMed]
- Godeau B, Caulier MT, Decuypere L, et al. Intravenous immunoglobulin for adults with autoimmune thrombocytopenic purpura: results of a randomized trial comparing 0.5 and 1 g/kg b.w. Br J Haematol 1999;107:716-9. [Crossref] [PubMed]
- Godeau B, Lesage S, Divine M, et al. Treatment of adult chronic autoimmune thrombocytopenic purpura with repeated high-dose intravenous immunoglobulin. Blood 1993;82:1415-21. [Crossref] [PubMed]
- Bussel J. Treatment of immune thrombocytopenic purpura in adults. Semin Hematol 2006;43:S3-10; discussion S8-9. [Crossref] [PubMed]
- Kurlander R, Coleman RE, Moore J, et al. Comparison of the efficacy of a two-day and a five-day schedule for infusing intravenous gamma globulin in the treatment of immune thrombocytopenic purpura in adults. Am J Med 1987;83:17-24. [Crossref] [PubMed]
- Julia A, Kovaleva L, Loria S, et al. Clinical efficacy and safety of Flebogammadif, a new high-purity human intravenous immunoglobulin, in adult patients with chronic idiopathic thrombocytopenic purpura. Transfus Med 2009;19:260-8. [Crossref] [PubMed]
- Dash CH, Gillanders KR, Stratford Bobbitt ME, et al. Safety and efficacy of Gammaplex(R) in idiopathic thrombocytopenic purpura (ClinicalTrials.gov--NCT00504075). PLoS One 2014;9:e96600 [Crossref] [PubMed]
- Newland AC, Burton I, Cavenagh JD, et al. Vigam-S, a solvent/detergent-treated intravenous immunoglobulin, in idiopathic thrombocytopenic purpura. Transfus Med 2001;11:37-44. [Crossref] [PubMed]
- Wietek S, Svorc D, Debes A, et al. Tolerability and safety of the intravenous immunoglobulin octagam(®) 10% in patients with immune thrombocytopenia: a post-authorisation safety analysis of two non-interventional phase IV trials. Hematology 2018;23:242-7. [Crossref] [PubMed]
- Moschese V, Canessa C, Trizzino A, et al. Pediatric subset of primary immunodeficiency patients treated with SCIG: post hoc analysis of SHIFT and IBIS pooled data. Allergy Asthma Clin Immunol 2020;16:80. [Crossref] [PubMed]
- Rodeghiero F, Woszczyk D, Slama B, et al. Efficacy and Safety of IQYMUNE(R), a Ten Percent Intravenous Immunoglobulin in Adult Patients With Chronic, Primary Immune Thrombocytopenia. J Hematol 2018;7:87-95. [Crossref] [PubMed]
- Apte S, Navarro-Puerto J, Damodar S, et al. Safety and efficacy of intravenous immunoglobulin (Flebogamma(®) 10% DIF) in patients with immune thrombocytopenic purpura. Immunotherapy 2019;11:81-9. [Crossref] [PubMed]
- Wasserman RL. Gammaplex(®) 5 and 10% in the treatment of primary immunodeficiency and chronic immune thrombocytopenic purpura. Immunotherapy 2017;9:1071-88. [Crossref] [PubMed]
- Bussel JB, Pham LC. Intravenous treatment with gammaglobulin in adults with immune thrombocytopenic purpura: review of the literature. Vox Sang 1987;52:206-11. [Crossref] [PubMed]
- Stasi R, Provan D. Management of immune thrombocytopenic purpura in adults. Mayo Clin Proc 2004;79:504-22. [Crossref] [PubMed]
- Bussel JB, Pham LC, Aledort L, et al. Maintenance treatment of adults with chronic refractory immune thrombocytopenic purpura using repeated intravenous infusions of gammaglobulin. Blood 1988;72:121-7. [Crossref] [PubMed]
- Puavilai T, Thadanipon K, Rattanasiri S, et al. Treatment efficacy for adult persistent immune thrombocytopenia: a systematic review and network meta-analysis. Br J Haematol 2020;188:450-9. [Crossref] [PubMed]
- Imbach P, Barandun S, d'Apuzzo V, et al. High-dose intravenous gammaglobulin for idiopathic thrombocytopenic purpura in childhood. Lancet 1981;1:1228-31. [Crossref] [PubMed]
- Imbach P, Morell A. Idiopathic thrombocytopenic purpura (ITP): immunomodulation by intravenous immunoglobulin (IVIg). Int Rev Immunol 1989;5:181-8. [Crossref] [PubMed]
- Bussel JB. Fc receptor blockade and immune thrombocytopenic purpura. Semin Hematol 2000;37:261-6. [Crossref] [PubMed]
- Salama A, Mueller-Eckhardt C, Kiefel V. Effect of intravenous immunoglobulin in immune thrombocytopenia. Lancet 1983;2:193-5. [Crossref] [PubMed]
- Berkman SA, Lee ML, Gale RP. Clinical uses of intravenous immunoglobulins. Semin Hematol 1988;25:140-58. [PubMed]
- Leong H, Stachnik J, Bonk ME, et al. Unlabeled uses of intravenous immune globulin. Am J Health Syst Pharm 2008;65:1815-24. [Crossref] [PubMed]
- Perez EE. Immunoglobulin use in immune deficiency and autoimmune disease states. Am J Manag Care 2019;25:S92-7. [PubMed]
- Samuelsson A, Towers TL, Ravetch JV. Anti-inflammatory activity of IVIG mediated through the inhibitory Fc receptor. Science 2001;291:484-6. [Crossref] [PubMed]
- Kaneko Y, Nimmerjahn F, Ravetch JV. Anti-inflammatory activity of immunoglobulin G resulting from Fc sialylation. Science 2006;313:670-3. [Crossref] [PubMed]
- Neschadim A, Branch DR. Mouse Models for Immune-Mediated Platelet Destruction or Immune Thrombocytopenia (ITP). Curr Protoc Immunol 2016;113:15.30.1-15.30.13.
- Crow AR, Song S, Semple JW, et al. IVIg inhibits reticuloendothelial system function and ameliorates murine passive-immune thrombocytopenia independent of anti-idiotype reactivity. Br J Haematol 2001;115:679-86. [Crossref] [PubMed]
- Branch DR. Unraveling the IVIG mystique. Transfusion 2013;53:242-4. [Crossref] [PubMed]
- Lewis BJB, Branch DR. Mouse Models of Rheumatoid Arthritis for Studies on Immunopathogenesis and Preclinical Testing of Fc Receptor-Targeting Biologics. Pharmacology 2020;105:618-29. [Crossref] [PubMed]
- Schwab I, Mihai S, Seeling M, et al. Broad requirement for terminal sialic acid residues and FcgammaRIIB for the preventive and therapeutic activity of intravenous immunoglobulins in vivo. Eur J Immunol 2014;44:1444-53. [Crossref] [PubMed]
- Anthony RM, Wermeling F, Karlsson MC, et al. Identification of a receptor required for the anti-inflammatory activity of IVIG. Proc Natl Acad Sci U S A 2008;105:19571-8. [Crossref] [PubMed]
- Anthony RM, Ravetch JV. A novel role for the IgG Fc glycan: the anti-inflammatory activity of sialylated IgG Fcs. J Clin Immunol 2010;30:S9-14. [Crossref] [PubMed]
- Anthony RM, Kobayashi T, Wermeling F, et al. Intravenous gammaglobulin suppresses inflammation through a novel T(H)2 pathway. Nature 2011;475:110-3. [Crossref] [PubMed]
- Leontyev D, Neschadim A, Branch DR. Cytokine profiles in mouse models of experimental immune thrombocytopenia reveal a lack of inflammation and differences in response to intravenous immunoglobulin depending on the mouse strain. Transfusion 2014;54:2871-9. [Crossref] [PubMed]
- Guhr T, Bloem J, Derksen NI, et al. Enrichment of sialylated IgG by lectin fractionation does not enhance the efficacy of immunoglobulin G in a murine model of immune thrombocytopenia. PLoS One 2011;6:e21246 [Crossref] [PubMed]
- Leontyev D, Katsman Y, Ma XZ, et al. Sialylation-independent mechanism involved in the amelioration of murine immune thrombocytopenia using intravenous gammaglobulin. Transfusion 2012;52:1799-805. [Crossref] [PubMed]
- Leontyev D, Katsman Y, Branch DR. Mouse background and IVIG dosage are critical in establishing the role of inhibitory Fcγ receptor for the amelioration of experimental ITP. Blood 2012;119:5261-4. [Crossref] [PubMed]
- Nagelkerke SQ, Dekkers G, Kustiawan I, et al. Inhibition of FcgammaR-mediated phagocytosis by IVIg is independent of IgG-Fc sialylation and FcgammaRIIb in human macrophages. Blood 2014;124:3709-18. [Crossref] [PubMed]
- von Gunten S, Shoenfeld Y, Blank M, et al. IVIG pluripotency and the concept of Fc-sialylation: challenges to the scientist. Nat Rev Immunol 2014;14:349. [Crossref] [PubMed]
- Crow AR, Lazarus AH. Mechanistic properties of intravenous immunoglobulin in murine immune thrombocytopenia: support for FcgammaRIIB falls by the wayside. Semin Hematol 2016;53:S20-2. [Crossref] [PubMed]
- Maddur MS, Stephen-Victor E, Das M, et al. Regulatory T cell frequency, but not plasma IL-33 levels, represents potential immunological biomarker to predict clinical response to intravenous immunoglobulin therapy. J Neuroinflammation 2017;14:58. [Crossref] [PubMed]
- Lewis BJB, Leontyev D, Neschadim A, et al. GM-CSF and IL-4 are not involved in IVIG-mediated amelioration of ITP in mice: a role for IL-11 cannot be ruled out. Clin Exp Immunol 2018;193:293-301. [Crossref] [PubMed]
- Temming AR, Dekkers G, van de Bovenkamp FS, et al. Human DC-SIGN and CD23 do not interact with human IgG. Sci Rep 2019;9:9995. [Crossref] [PubMed]
- Clarkson SB, Bussel JB, Kimberly RP, et al. Treatment of refractory immune thrombocytopenic purpura with an anti-Fc gamma-receptor antibody. N Engl J Med 1986;314:1236-9. [Crossref] [PubMed]
- Bussel J, Patel V, Dunbar C, et al. GMA161 Treatment of Refractory ITP: Efficacy of Fcγ-RIII Blockade. Blood 2006;108:1074. [Crossref]
- Nakar C, Bussel J. 3G8 and GMA161, Anti-FcγRIII Inhibitory Monoclonal Antibodies in the Treatment of Chronic Refractory ITP. (Summary of 2 Pilot Studies). Blood 2009;114:2404. [Crossref]
- Peter AAN, George BS, Burack WR, et al. FcγRI and FcγRIII on splenic macrophages mediate phagocytosis of anti-glycoprotein IIb/IIIa autoantibody-opsonized platelets in immune thrombocytopenia. Haematologica 2020; [Crossref]
- Lewis BJ. The Role of Modified Fc Fragments in Treating Autoimmune Diseases: A Potential Replacement for Intravenous Immunoglobulin [Doctoral dissertation]: University of Toronto; 2020.
- Katsman Y, Foo AH, Leontyev D, et al. Improved mouse models for the study of treatment modalities for immune-mediated platelet destruction. Transfusion 2010;50:1285-94. [Crossref] [PubMed]
- Barsam SJ, Psaila B, Forestier M, et al. Platelet production and platelet destruction: assessing mechanisms of treatment effect in immune thrombocytopenia. Blood 2011;117:5723-32. [Crossref] [PubMed]
- Meyer O, Winter O, Salama A. Influence of Intravenous Immunoglobulin Treatment on Thrombopoiesis. Transfus Med Hemother 2012;39:217-20. [Crossref] [PubMed]
- Putoczki T, Ernst M. More than a sidekick: the IL-6 family cytokine IL-11 links inflammation to cancer. J Leukoc Biol 2010;88:1109-17. [Crossref] [PubMed]
- Figueiredo CA, Drohomyrecky PC, McCarthy SD, et al. Optimal attenuation of experimental autoimmune encephalomyelitis by intravenous immunoglobulin requires an intact interleukin-11 receptor. PLoS One 2014;9:e101947 [Crossref] [PubMed]
- Vadhan-Raj S. Management of chemotherapy-induced thrombocytopenia: current status of thrombopoietic agents. Semin Hematol 2009;46:S26-32. [Crossref] [PubMed]
- Ciurea SO, Hoffman R. Cytokines for the treatment of thrombocytopenia. Semin Hematol 2007;44:166-82. [Crossref] [PubMed]
- Bussel JB, Mukherjee R, Stone AJ. A pilot study of rhuIL-11 treatment of refractory ITP. Am J Hematol 2001;66:172-7. [Crossref] [PubMed]
- Lin Y, Zhou X, Guo W, et al. RhIL-11 treatment normalized Th1/Th2 and T-bet/GATA-3 imbalance in in human immune thrombocytopenic purpura (ITP). Int Immunopharmacol 2016;38:40-4. [Crossref] [PubMed]
- Wu X, Wang L, Sun L, et al. Analysis of clinical effects and mechanism of recombinant human interleukin-11 with glucocorticoids for treatment of idiopathic thrombocytopenic purpura. Exp Ther Med 2017;13:519-22. [Crossref] [PubMed]
- Durandy A, Kaveri SV, Kuijpers TW, et al. Intravenous immunoglobulins--understanding properties and mechanisms. Clin Exp Immunol 2009;158:2-13. [Crossref] [PubMed]
- Ballow M. Mechanisms of immune regulation by IVIG. Curr Opin Allergy Clin Immunol 2014;14:509-15. [Crossref] [PubMed]
- Norris PAA, Kaur G, Lazarus AH. New insights into IVIg mechanisms and alternatives in autoimmune and inflammatory diseases. Curr Opin Hematol 2020;27:392-8. [Crossref] [PubMed]
- Yoshida Y. Historical review. The light and shadow of Paul Kaznelson: his life and contribution to hematology. Ann Hematol 2008;87:877-9. [Crossref] [PubMed]
- Coon WW. Splenectomy for idiopathic thrombocytopenic purpura. Surg Gynecol Obstet 1987;164:225-9. [PubMed]
- Lakshmanan S, Cuker A. Contemporary management of primary immune thrombocytopenia in adults. J Thromb Haemost 2012;10:1988-98. [Crossref] [PubMed]
- Mahévas M, Michel M, Godeau B. How we manage immune thrombocytopenia in the elderly. Br J Haematol 2016;173:844-56. [Crossref] [PubMed]
- Bylsma LC, Fryzek JP, Cetin K, et al. Systematic literature review of treatments used for adult immune thrombocytopenia in the second-line setting. Am J Hematol 2019;94:118-32. [Crossref] [PubMed]
- Al-Samkari H, Kuter DJ. Immune Thrombocytopenia in Adults: Modern Approaches to Diagnosis and Treatment. Semin Thromb Hemost 2020;46:275-88. [Crossref] [PubMed]
- Jaime-Pérez JC, Aguilar-Calderon P, Jimenez-Castillo RA, et al. Treatment outcomes and chronicity predictors for primary immune thrombocytopenia: 10-year data from an academic center. Ann Hematol 2020;99:2513-20. [Crossref] [PubMed]
- Dou X, Yang R. Current and emerging treatments for immune thrombocytopenia. Expert Rev Hematol 2019;12:723-32. [Crossref] [PubMed]
- Ahn YS, Harrington WJ. Treatment of idiopathic thrombocytopenic purpura (ITP). Annu Rev Med 1977;28:299-309. [Crossref] [PubMed]
- Rodeghiero F. First-line therapies for immune thrombocytopenic purpura: re-evaluating the need to treat. Eur J Haematol Suppl 2008;19-26. [Crossref] [PubMed]
- Bagrodia N, Button AM, Spanheimer PM, et al. Morbidity and mortality following elective splenectomy for benign and malignant hematologic conditions: analysis of the American College of Surgeons National Surgical Quality Improvement Program data. JAMA Surg 2014;149:1022-9. [Crossref] [PubMed]
- Gonzalez-Porras JR, Escalante F, Pardal E, et al. Safety and efficacy of splenectomy in over 65-yrs-old patients with immune thrombocytopenia. Eur J Haematol 2013;91:236-41. [Crossref] [PubMed]
- Ghanima W, Godeau B, Cines DB, et al. How I treat immune thrombocytopenia: the choice between splenectomy or a medical therapy as a second-line treatment. Blood 2012;120:960-9. [Crossref] [PubMed]
- Tahir F, Ahmed J, Malik F. Post-splenectomy Sepsis: A Review of the Literature. Cureus 2020;12:e6898 [Crossref] [PubMed]
- Ingawale DK, Mandlik SK. New insights into the novel anti-inflammatory mode of action of glucocorticoids. Immunopharmacol Immunotoxicol 2020;42:59-73. [Crossref] [PubMed]
- Dameshek W, Rubio F Jr, Mahoney JP, et al. Treatment of idiopathic thrombocytopenic purpura (ITP) with prednisone. J Am Med Assoc 1958;166:1805-15. [Crossref] [PubMed]
- Fujimura K. Glucocorticoids therapy as a first line treatment in ITP. Nihon Rinsho 2003;61:593-8. [PubMed]
- Borst F, Keuning JJ, van Hulsteijn H, et al. High-dose dexamethasone as a first- and second-line treatment of idiopathic thrombocytopenic purpura in adults. Ann Hematol 2004;83:764-8. [Crossref] [PubMed]
- Kubasch AS, Kisro J, Hessling J, et al. Disease management of patients with immune thrombocytopenia-results of a representative retrospective survey in Germany. Ann Hematol 2020;99:2085-93. [Crossref] [PubMed]
- Mizutani H, Furubayashi T, Imai Y, et al. Mechanisms of corticosteroid action in immune thrombocytopenic purpura (ITP): experimental studies using ITP-prone mice, (NZW x BXSB) F1. Blood 1992;79:942-7. [Crossref] [PubMed]
- Alan IS, Alan B. Side effects of glucocorticoids. Pharmacokinetics and Adverse Effects of Drugs-Mechanisms and Risks Factors. IntechOpen; 2018. doi:
10.5772/intechopen.72019 . Available online: https://www.intechopen.com/books/pharmacokinetics-and-adverse-effects-of-drugs-mechanisms-and-risks-factors/side-effects-of-glucocorticoids - Samson M, Fraser W, Lebowitz D. Treatments for Primary Immune Thrombocytopenia: A Review. Cureus 2019;11:e5849 [Crossref] [PubMed]
- Imbach P. Treatment of immune thrombocytopenia with intravenous immunoglobulin and insights for other diseases. A historical review. Swiss Med Wkly 2012;142:w13593 [PubMed]
- Rodeghiero F, Marranconi E. Management of immune thrombocytopenia in women: current standards and special considerations. Expert Rev Hematol 2020;13:175-85. [Crossref] [PubMed]
- Marie I, Maurey G, Herve F, et al. Intravenous immunoglobulin-associated arterial and venous thrombosis; report of a series and review of the literature. Br J Dermatol 2006;155:714-21. [Crossref] [PubMed]
- Jin PH, Shin SC, Dhamoon MS. Risk of thrombotic events after inpatient intravenous immunoglobulin or plasma exchange for neurologic disease: A case-crossover study. Muscle Nerve 2020;62:327-32. [Crossref] [PubMed]
- Pendergrast J, Binnington B, Tong TN, et al. Incidence and Risk Factors for IVIG-Mediated Hemolysis. Blood 2017;130:2398.
- Michelis FV, Branch DR, Scovell I, et al. Acute hemolysis after intravenous immunoglobulin amid host factors of ABO-mismatched bone marrow transplantation, inflammation, and activated mononuclear phagocytes. Transfusion 2014;54:681-90. [Crossref] [PubMed]
- Hoppe I. Antibody screening of commercially available immunoglobulins. Erythrocyte-, HLA- and autoantibodies (author's transl). Blut 1979;39:9-16. [Crossref] [PubMed]
- Kluge A, Dopfer R, Pfeiffer-Wolf I, et al. Immunoglobulin high-dose therapy: RBC-alloantibodies in commercial preparations and haemolytic anaemia: a case report. Beitr Infusionsther Transfusionsmed 1994;32:474-5. [PubMed]
- Becker T, Küenzlen E, Salama A, et al. Treatment of childhood idiopathic thrombocytopenic purpura with Rhesus antibodies (anti-D). Eur J Pediatr 1986;145:166-9. [Crossref] [PubMed]
- Smith NA, Chakraverty RK, Boughton BJ. The successful treatment of idiopathic thrombocytopenic purpura with the low dose non specific IgG component of anti D immunoglobulin. Clin Lab Haematol 1990;12:131-6. [Crossref] [PubMed]
- Scaradavou A, Woo B, Woloski BM, et al. Intravenous anti-D treatment of immune thrombocytopenic purpura: experience in 272 patients. Blood 1997;89:2689-700. [Crossref] [PubMed]
- Olofinboba KA, Greenberg BR. Successful treatment of infectious mononucleosis-associated immune thrombocytopenia with WinRho anti-D immunoglobulin complicated by severe hemolysis. Am J Hematol 2000;65:178. [Crossref] [PubMed]
- Levendoglu-Tugal O, Jayabose S. Intravenous anti-D immune globulin-induced intravascular hemolysis in Epstein-Barr virus-related thrombocytopenia. J Pediatr Hematol Oncol 2001;23:460-3. [Crossref] [PubMed]
- Rewald MD, Francischetti MM. After eight-year-tolerance minimal i.v. anti-D infusions unleash hemolysis in a patient with immune thrombocytopenic purpura (ITP). Transfus Apher Sci 2004;30:105-10. [Crossref] [PubMed]
- Gaines AR. Disseminated intravascular coagulation associated with acute hemoglobinemia or hemoglobinuria following Rh(0)(D) immune globulin intravenous administration for immune thrombocytopenic purpura. Blood 2005;106:1532-7. [Crossref] [PubMed]
- Wang X, Xu Y, Gui W, et al. Retrospective analysis of different regimens for Chinese adults with severe newly diagnosed immune thrombocytopenia. Clin Exp Med 2020;20:381-5. [Crossref] [PubMed]
- Artru F, Alberio L, Moradpour D, et al. Acute immune thrombocytopaenic purpura in a patient with COVID-19 and decompensated cirrhosis. BMJ Case Rep 2020;13:e236815 [Crossref] [PubMed]
Cite this article as: Almizraq RJ, Branch DR. Efficacy and mechanism of intravenous immunoglobulin treatment for immune thrombocytopenia in adults. Ann Blood 2021;6:2.