Evaluating “treatment as prevention” on the road to hepatitis C virus elimination
Fraser and colleagues (1) have recently published the results of a study modelling the direct acting antiviral (DAA) scale-up requirements needed to reduce hepatitis C virus (HCV) infection in people who inject drugs (PWID) within selected European sites. Such “treatment as prevention” strategies have been previously proposed to address the ambitious WHO HCV elimination target for 2030 (2), established in response to the world-wide exponential growth in HCV-associated liver disease which has occurred in the past few decades and is a leading cause of increased liver-related mortality (3). Although a challenging proposal even for resource-rich regions such as Europe, the WHO target is now deemed feasible with the advent of DAA treatments which achieve sustained viral response (SVR) rates above 90% for the most prevalent genotypes (4). As illustrated through the modelling of comparative interventions, Fraser et al. (1) conclude that “treatment as prevention” is a viable strategy for curtailing the future burden of disease. Although transmission of HCV occurs primarily among PWID in most resource-rich countries, treatment recommendation guidelines have historically excluded PWID because of concerns surrounding reinfection rates and compliance to treatment regimens involving pegylated interferon which can have significant side effects. However, current European, AASLD/IDSA and WHO treatment guidelines indicate a reversal in this position owing to the relative ease of treatment with DAAs and evidence that high rates of SVR are achievable among this group (5,6). The prioritisation of PWID for treatment is recognised as a potentially effective strategy to significantly decrease HCV incidence by not only reducing the prevalence of existing chronic cases of HCV infection but additionally preventing new transmission events (7). However, the likely effectiveness and long-term epidemiological impact of wider access to treatment alongside existing harm reduction prevention strategies is not well understood.
In order to gain a more comprehensive understanding of the scale-up of DAA treatment required to reduce HCV infection in PWID to minimal levels for 11 European sites, Fraser et al. (1) have developed a deterministic mathematical model parameterised using published data from each of the geographical sites. In addition to treatment status, the model was stratified according to opioid substitution therapy (OST) and needle and syringe programmes (NSPs) allowing for different infection rates among these groups. Results suggest that treatment scale-up will be needed to reduce HCV prevalence in all but three of the sites. Only modest reductions in prevalence are estimated to be achieved in Belgium, Denmark, Hamburg, Norway and Scotland by doubling the current DAA treatment rates and at least a fivefold increase in the current HCV treatment rates will be needed to reduce HCV incidence to <2% by 2026. Due to the high prevalence of chronic HCV in Finland and Sweden, treatment rates of 50/1,000 PWID will be required to halve prevalence by 2026. Increasing OST and NSP coverage to 80% whilst maintaining current treatment rates is projected to reduce prevalence by at least 30% in all sites except Finland and Hamburg. However, the relative benefit of PWID-targeted treatment allocation and traditional harm reduction preventative measures was difficult to ascertain owing to uncertainty in highly influential model parameters such as injection duration. Nevertheless, this study identifies important knowledge gaps that must be confronted if optimal and realistic public health policy decisions are to be determined and implemented.
Quantitative models play an increasingly important role in guiding public health policy decisions, through improved understanding of infection transmission dynamics and forecasting the impact of interventions on infection incidence and disease burden. Several studies, employing methods such as deterministic mathematical modelling and stochastic simulations, have evaluated the impact of varying treatment rates and PWID-targeted allocation strategies on HCV infection prevalence and/or the burden of severe liver morbidity (8-10). These studies have focused on generalised or single country settings. However, the spread of HCV among PWID is known to occur across broad geographical scales, with inter-country spread (11) and the potential for multiple independent virus introduction events (12). Given variation in critical features of HCV epidemiology and treatment delivery between geographically disperse regions, the impact of PWID-targeted treatment is not expected to be uniform globally. A concerted transboundary effort, with treatment interventions appraised at both local and international scales, will be essential on the road to global HCV elimination.
Broad-scale deterministic models, such as those used by Fraser et al. (1), provide a flexible framework for comparing general scenarios for multiple regions in the face of sparse data. However, these models are not designed for accurate predictions which require a high degree of confidence in empirically-driven model assumptions. Additional sources of epidemiological variation compounds accurate predictions of treatment impact by country.
Firstly, the geographical distribution of HCV genotypes and subtypes is known to vary within and between continents, with large-scale movement of HCV among PWID recognised within Europe (11,13). Consequently, the relative rollout of different DAA therapy combinations and treatment durations could vary geographically. Furthermore, despite the advent of second-generation DAAs with pan-genotypic action, clinical trials have shown drug efficacy can vary between HCV genotypes and subtypes (4). However, extensive observational data accrued through long-term routine clinical usage is needed to fully elucidate the genotype-specific effectiveness of DAAs in the real-world setting. The temporal and spatial dynamics of HCV transmission and associated treatment practices must therefore be borne in mind to ensure predictive models of treatment impacts are timely, relevant, and of public health utility.
Secondly, deterministic mathematical models in this context make the unrealistic assumption of random contact patterns among PWID. The sharing of needles and syringes are the major empirically supported source of exposure for this group (14). The network of contacts among PWID via this route is characterised by a non-homogeneous pattern, with transmission events being more likely among affiliates of certain subpopulations. For example, several studies utilising virus sequences have found a high degree of phylogenetic clustering of HCV infections, and these clusters have discernible shared biological characteristics such as recent seroconversion or HIV co-infection (15). The genetic clustering of HCV among HIV co-infected PWID is particularly significant considering the higher HCV RNA levels associated with HIV co-infection (16), suggesting a greater chance of transmission from these individuals. Indeed, a modelling study investigating the targeting of treatment at HIV/HCV co-infected individuals has shown this to be an effective strategy in reducing transmission (17).
Social components of HCV transmission among PWID have also been studied and highlight the potential for particularly prominent individuals that may act as infection ‘super spreaders’ (18). Another important component of PWID networks is incarceration, which presents an independent and high risk environment for the generation of new HCV infections as well as the increased chance of re-infection (19). Phylogeographic studies have also revealed a highly localised spatial clustering component to HCV transmission providing further insights into potential demographic, social, or economically-driven transmission hotspots which could be exploited for targeting treatment interventions (12). As well as social network features suggestive of heightened transmission risk, other behavioural factors may provide protective outcomes. For example, community-based surveillance has identified acts of “serosorting”, whereby HCV positive persons preferentially share injection equipment with others of known HCV positive status (20). Such behaviours may limit the occurrence of transmission among PWID networks. Further studies incorporating non-random contact structures [see (21) for an example of an individual-based stochastic modelling of HCV transmission among PWID] may improve the utility of models evaluating “treatment as prevention”.
Whilst DAA treatment is likely to greatly reduce the prevalence of HCV in the PWID population, resistance-associated substitutions (RAS) can emerge in individuals who do not attain an SVR. A single mutation is frequently sufficient to induce antiviral resistance in some DAAs that have a low barrier to resistance and, although HCV strains containing RAS tend to be less robust than their wild type counterparts, the evolution of compensatory mutations can improve fitness to wild type levels. To prevent the emergence of resistance, DAAs are generally given as a combination therapy consisting of two or more drugs. Despite this however resistant strains do occur in a small number of individuals. If treatment for actively injecting PWID is implemented, it is possible that HCV strains with established RAS from the small number of individuals failing treatment will be transmitted to others, setting the stage for infection and re-infection of the PWID population with drug-resistant strains through the sharing of injecting equipment. Infection with HCV strains that carry RAS need not be dependent on the emergence and transmission of HCV strains with compensatory mutations; it is recognised that transmitted antiretroviral resistance associated with low fitness HIV variants occurs, albeit at a reduced transmission frequency compared to wild type HIV (22). In addition to RAS emergence, there are genotypes and sub-genotypes that display an inherent resistance to certain DAAs, such as HCV subtypes gt1l, gt4r and subtypes of gt3 (23,24). These subtypes are not widely distributed across PWID populations in Europe but a marked reduction in the frequency of the predominant sub-genotypes could create a niche suitable for the expansion of alternative strains which are unable to out-compete current dominant strains either due to replication and transmission fitness or through geographical restrictions. In the advent of universal DAA treatment for actively injecting individuals it will be of the utmost importance to ensure strategies to monitor and limit the emergence of antiviral resistant HCV strains are firmly in place.
Although many important challenges remain, such as the delivery of treatment to marginalised groups including PWID and the potential for the emergence and spread of RAS, the targeting of treatment to people at high risk of transmitting HCV will likely be key to the successful global elimination of HCV as a public health concern. Given geographical variability in genotype distribution and the role of social and spatial networks in the spread of HCV, consideration of PWID networks and transmission hotspots may prove imperative in the design of optimal treatment allocation strategies and the monitoring of emergent strains carrying RAS. The incorporation of contact structure is a prudent aim for future modelling exercises, for accurate, context-specific, predictions of the impact of different treatment rates and allocation strategies (Figure 1). Ultimately, model effectiveness will remain difficult in the absence of good quality data and improved health monitoring infrastructure in some settings; ongoing surveillance of PWID populations will be critical in this endeavour (25).
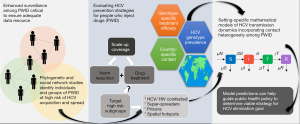
Acknowledgments
Funding: EC McWilliam Leitch and J McLauchlan were supported by the Medical Research Council (MRC) award MC_UU_12014/1. S Nickbakhsh was supported by MRC award MC_UU_12014/9.
Footnote
Provenance and Peer Review: This article was commissioned and reviewed by the Section Editor Dr. Ru Xu (Guangzhou Blood Center, Guangzhou, China).
Conflicts of Interest: All authors have completed the ICMJE uniform disclosure form (available at http://dx.doi.org/10.21037/aob.2018.10.05). The authors have no conflicts of interest to declare.
Ethical Statement: The authors are accountable for all aspects of the work in ensuring that questions related to the accuracy or integrity of any part of the work are appropriately investigated and resolved.
Open Access Statement: This is an Open Access article distributed in accordance with the Creative Commons Attribution-NonCommercial-NoDerivs 4.0 International License (CC BY-NC-ND 4.0), which permits the non-commercial replication and distribution of the article with the strict proviso that no changes or edits are made and the original work is properly cited (including links to both the formal publication through the relevant DOI and the license). See: https://creativecommons.org/licenses/by-nc-nd/4.0/.
References
- Fraser H, Martin NK, Brummer-Korvenkontio H, et al. Model projections on the impact of HCV treatment in the prevention of HCV transmission among people who inject drugs in Europe. J Hepatol 2018;68:402-11. [Crossref] [PubMed]
- WHO. Combating hepatitis B and C to reach elimination by 2030. Available online: http://apps.who.int/iris/bitstream/handle/10665/206453/WHO_HIV_2016.04_eng.pdf?sequence=1
- Polaris Observatory HCV Collaborators. Global prevalence and genotype distribution of hepatitis C virus infection in 2015: a modelling study. Lancet Gastroenterol Hepatol 2017;2:161-76. [Crossref] [PubMed]
- Lawitz E, Mangia A, Wyles D, et al. Sofosbuvir for previously untreated chronic hepatitis C infection. N Engl J Med 2013;368:1878-87. [Crossref] [PubMed]
- AASLD/IDSA. HCV Guidance: Recommendations for Testing, Managing, and Treating Hepatitis C. Available online: https://www.hcvguidelines.org/unique-populations/pwid
- European Association for the Study of the Liver. EASL Recommendations on Treatment of Hepatitis C 2016. J Hepatol 2017;66:153-94. [Crossref] [PubMed]
- Hellard M, Doyle JS, Sacks-Davis R, et al. Eradication of Hepatitis C Infection: The Importance of Targeting People Who Inject Drugs. Hepatology 2014;59:366-9. [Crossref] [PubMed]
- Innes H, Goldberg D, Dillon J, et al. Strategies for the treatment of Hepatitis C in an era of interferon-free therapies: what public health outcomes do we value most? Gut 2015;64:1800-9. [Crossref] [PubMed]
- Harris RJ, Thomas B, Griffiths J, et al. Increased uptake and new therapies are needed to avert rising hepatitis C-related end stage liver disease in England: modelling the predicted impact of treatment under different scenarios. J Hepatol 2014;61:530-7. [Crossref] [PubMed]
- Martin NK, Vickerman P, Foster GR, et al. Can antiviral therapy for hepatitis C reduce the prevalence of HCV among injecting drug user populations? A modeling analysis of its prevention utility. J Hepatol 2011;54:1137-44. [Crossref] [PubMed]
- van Asten L, Verhaest I, Lamzira S, et al. Spread of hepatitis C virus among European injection drug users infected with HIV: a phylogenetic analysis. J Infect Dis 2004;189:292-302. [Crossref] [PubMed]
- McNaughton AL, Cameron ID, Wignall-Fleming EB, et al. Spatiotemporal Reconstruction of the Introduction of Hepatitis C Virus into Scotland and Its Subsequent Regional Transmission. J Virol 2015;89:11223-32. [Crossref] [PubMed]
- Messina JP, Humphreys I, Flaxman A, et al. Global distribution and prevalence of hepatitis C virus genotypes. Hepatology 2015;61:77-87. [Crossref] [PubMed]
- Miller ER, Hellard ME, Bowden S, et al. Markers and risk factors for HCV, HBV and HIV in a network of injecting drug users in Melbourne, Australia. J Infect 2009;58:375-82. [Crossref] [PubMed]
- Jacka B, Applegate T, Krajden M, et al. Phylogenetic clustering of hepatitis C virus among people who inject drugs in Vancouver, Canada. Hepatology 2014;60:1571-80. [Crossref] [PubMed]
- Thomas DL, Astemborski J, Vlahov D, et al. Determinants of the quantity of hepatitis C virus RNA. J Infect Dis 2000;181:844-51. [Crossref] [PubMed]
- Bartlett SR, Wertheim JO, Bull RA, et al. A molecular transmission network of recent hepatitis C infection in people with and without HIV: Implications for targeted treatment strategies. J Viral Hepat 2017;24:404-11. [Crossref] [PubMed]
- Wang Y, Tan XD, Zhou C, et al. Exploratory social network analysis and gene sequencing in people who inject drugs infected with hepatitis C virus. Epidemiol Infect 2016;144:3080-90. [Crossref] [PubMed]
- Bate JP, Colman AJ, Frost PJ, et al. High prevalence of late relapse and reinfection in prisoners treated for chronic hepatitis C. Journal of Gastroenterology and Hepatology 2010;25:1276-80. [Crossref] [PubMed]
- Smith BD, Jewett A, Burt RD, et al. "To share or not to share?" Serosorting by hepatitis C status in the sharing of drug injection equipment among NHBS-IDU2 participants. J Infect Dis 2013;208:1934-42. [Crossref] [PubMed]
- Rolls DA, Daraganova G, Sacks-Davis R, et al. Modelling hepatitis C transmission over a social network of injecting drug users. J Theor Biol 2012;297:73-87. [Crossref] [PubMed]
- Leigh Brown AJ, Frosrt SD, Mathews WC, et al. Transmission fitness of drug-resistant human immunodeficiency virus and the prevalence of resistance in the antiretroviral-treated population. J Infect Dis 2003;187:683-6. [Crossref] [PubMed]
- da Silva Filipe A, Sreenu V, Hughes J, et al. Response to DAA therapy in the NHS England Early Access Programme for rare HCV subtypes from low and middle income countries. J Hepatol 2017;67:1348-50. [Crossref] [PubMed]
- Smith D, Magri A, Bonsall D, et al. Resistance analysis of genotype 3 hepatitis C virus indicates subtypes inherently resistant to nonstructural protein 5A inhibitors. Hepatology 2018; [Epub ahead of print]. [Crossref] [PubMed]
- Hickman M, De Angelis D, Vickerman P, et al. HCV Treatment as Prevention in People Who Inject Drugs - testing the evidence. Curr Opin Infect Dis 2015;28:576-82. [Crossref] [PubMed]
Cite this article as: Nickbakhsh S, McLauchlan J, McWilliam Leitch EC. Evaluating “treatment as prevention” on the road to hepatitis C virus elimination. Ann Blood 2018;3:44.